Mimicking mussels’ muscle
Shellfish ‘teach’ researchers how to make superglues for underwater uses — or even to bind wounds better than stitches
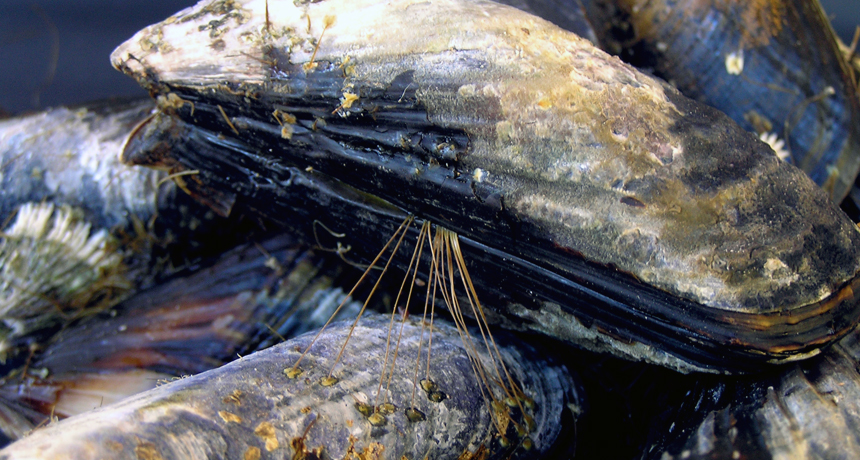
Mussels hold fast to hard surfaces, including other mussel shells, with the help of dozens of fibers called byssal threads and multiple superglues, all self-made.
University of Washington/Carrington Lab
By Sid Perkins
The strongest creatures at the beach aren’t muscle-bound bodybuilders. To find these marvels, you actually need to look near the waterline. There, waves pound the shore mercilessly and the tides slosh in and out twice a day. Tucked amongst the rocks, clinging tightly, you’ll find shellfish called mussels.
“These little creatures live in a hostile environment simply by hunkering down and holding on to hard surfaces,” observes J. Herbert Waite. He works at the University of California, Santa Barbara. As a molecular biologist, he studies the processes that happen inside living cells.
Some of the organisms he studies are mussels, a type of dark-shelled bivalve. Having no backbone, these invertebrates live inside two shells that can clamp together for protection. Some bivalves live in seafloor mud. Many others burrow into wood that’s submerged. Regardless of their lifestyle, most bivalves filter their food from the water that constantly flows past them.
But among bivalves, mussels are special, says Waite. “They have a heroic ability to stick to wet surfaces.”
And research suggests that science can learn a lot from mussels. The fibers they make to anchor themselves to hard surfaces are seven times stretchier than any fibers made by people. In fact, the filaments are so stretchy that they’ve been called “nature’s bungee cords.” The goo that mussels exude to cement the fibers to hard surfaces works wonders, too. It helps mussels stick around even in wet conditions, no matter if the liquid is as salty as the ocean or as fresh as lake water.
These unusual fibers and adhesive have captured the attention of materials scientists. The researchers are looking to adopt some of the mussel’s tricks to make new glues that will work even on things that are wet.
For instance, human blood is salty like the sea. This suggests a mussel-like glue might work on and in the body, such as to close wounds.
Yet even as researchers look for help from mussels to design new superglues, people may be causing some of these same animals to lose their grip. The global warming fostered by people’s extensive use of fossil fuels could be harming the natural anchors that mussels make.
Holding tight
Coastlines are rough environments. Organisms that can’t attach themselves to rocks, docks or other solid objects will get tossed around by the constantly shifting currents. Scientists study the glues produced by mussels because they hold tight even in hostile seas, says Waite. But working in a wet world is not their only challenge. Mussel glues also hang on to surfaces that may be corroded or even covered with slime (known as biofilms). Those gooey films are made by bacteria and other microbes.
Although scientists would like to mimic mussel-made glues and stretchy proteins, their chemistry is “complex and messy,” says Waite. Take those natural bungee cords; scientists refer to these as byssal fibers. A single mussel needs dozens to stay put.

Each ingredient would normally dissolve in water. But inside the tube, chemical reactions take place that turn those ingredients into materials that are insoluble. (A fiber that dissolved in water wouldn’t hold down a mussel for long, would it?)
Waite has been studying mussels and the adhesives they make for more than 30 years. His teams, and many other scientists, have turned up interesting substances in the fibers. Some, very aptly, are called mussel foot proteins, or MFPs. Scientists have assigned each of these proteins a different number. For instance, MFP-1 is typically found in the outer surface of a fiber. Peptides and iron atoms within MFP-1 are chemically linked into long chains.
One of the more interesting foot proteins usually develops where a byssal fiber attaches to a hard surface. This MFP-5 is “an adhesive gorilla” — one of the strongest protein glues known, says Waite. Just 5 minutes after a mussel makes it, this glue has already reached 80 percent of its full strength.
Recently, experiments by Waite and his coworkers have shed new light on how three mussel foot proteins — MFP-1, MFP-3, and MFP-5 — bond to surfaces tainted with microbial films. Previous tests showed these films could be either hydrophobic (water-repellent) or hydrophilic (water-attracting). Which one depends on of the film’s microbes. The new tests show that under certain conditions, some mussel proteins stick better than others. All together, they enable mussels to stick to virtually any type of surface. These test results will help scientists design better chemical adhesives, they suggest in a paper published online Sept. 6 in Proceedings of the National Academy of Sciences.
By studying mussel foot proteins, Waite’s group hopes to develop synthetic glues that work better than those available today — and perhaps ones that cost less too.
Mussel medicine
Surgeons are among the experts who could benefit from adhesives that grip when wet. For instance, mussel-inspired glues could help securely join one part of an artificial hip joint to a leg bone. Or, dentists might cement an artificial crown onto a tooth.
The new glues could overcome one major limitation of current medical adhesives, says Phillip Messersmith. “Under wet conditions, such materials don’t hold very well or very long.” Messersmith is a biomedical engineer at Northwestern University in Evanston, Ill. He studies how materials, including ones naturally made by organisms, might be used for medical purposes.
The appeal of mussel-inspired adhesives is that they work in wet, salty environments — such as blood. They also will adhere to all sorts of materials, from tissues (such as flesh and bone) to manufactured materials (such as plastic and steel).
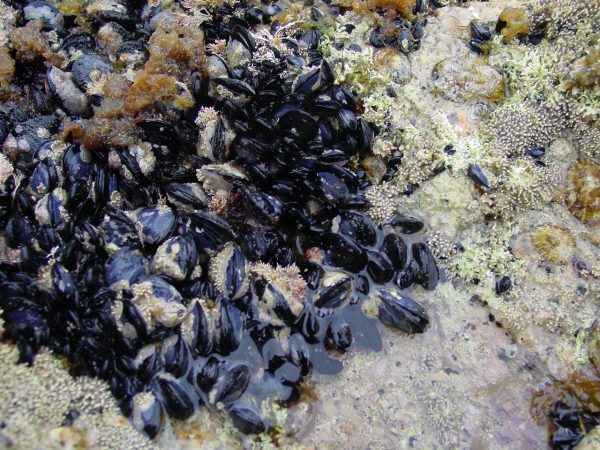
But his lab tests suggest that a mussel-inspired adhesive might prevent or minimize several problems with such fetal surgery. Besides bonding well to tissues, the glue can seal a hole about 3.5 millimeters (0.14 inch, or slightly more than 1/8 inch) across. That’s about the size that a tiny surgical instrument might make. Moreover, artificial glues can inflame tissue. The Northwestern team hopes that mussel-inspired substitutes might avoid this problem. Finally, a mussel glue could be designed to shrink as it warms to body temperature, Messersmith says. That would tend to pull tissues together and possibly speed healing. Right now, he notes, all adhesives and sealants currently approved for use inside the body swell after they’re applied.
In tests with pregnant rabbits, Messersmith and his coworkers cut a 2.1-millimeter (0.08 inch) hole in the amniotic sacs surrounding 46 fetuses. Where membranes were not sealed shut (which left a fetus vulnerable to infection), only 35 percent of the fetuses survived. But if a mussel adhesive was used to glue a patch of tissue over the cuts, about 80 percent of the fetuses survived.
Challenges still remain, says Messersmith. For instance, “We need to design a better device to deliver the sealant” to internal wound sites, he says. “But that’s an engineering problem,” he adds, suggesting that it shouldn’t be too hard to fix.
Warmer, weaker
Mussels, themselves, are confronting an engineering problem that could prove far more challenging. In tidal zones, the mussels anchored onto rocks provide food and habitat for many other creatures, notes biologist Emily Carrington. She works at the University of Washington in Friday Harbor, where she studies tidal communities. Her work has lately found signs that mussels may be in big trouble. The reason: climate change.
Mussels often crowd together so thickly that you can’t see the near-shore rocks they’re clinging to. But byssal fibers don’t last forever. Mussels must replace the fibers that break or wear out. Carrington’s lab tests now show that not all fibers are created equal. Ones made during summer are definitely weaker than those made at any other time of the year.
Fibers made between January and May last about two months, she finds. In contrast, those formed in the summer may last as little as two to three weeks.
When Carrington and her coworkers probed why, they found temperature plays a big role. Mussels living in waters that are 10° to 18° Celsius (50° to 64° Fahrenheit), produce strong and very elastic fibers. But when the water climbs to 25 °C, the scientists found, newly made fibers prove far less stretchy and less than half as strong.
Climate change is making coastal waters in many areas warmer. That means mussels should increasingly have a tough time maintaining their grip.
Much of Earth’s slowly rising temperature is driven by all of the carbon dioxide spewed by the burning of fossil fuels. Global warming would be climbing faster if the oceans hadn’t started sopping up some of that gas from the atmosphere. But one byproduct of the ocean’s absorption of this gas has been to make seawater more acidic.
As human activity sends more carbon dioxide into the atmosphere, more of that gas ends up dissolving in the oceans. That, in turn, makes the seas more acidic. In research published in March in Nature Climate Change, Carrington and her team showed water acidity also affects fiber strength. More acidic water weakens fibers. That’s a big problem, since scientists predict ocean acidity will increase even more in years to come.
The acid test
In lab experiments, Carrington’s team tested the strength of mussel fibers formed in waters with different pHs. A pH is a measure of water’s acidity. Each water sample’s pH was held at a different, specific number between 7.5 and 8. Waters with these pHs are slightly alkaline (a pH of 7 is neutral and anything below that is acidic). All of the test pHs were representative of seawater.
As the water’s acidity grew — meaning its pH fell — the strength of a mussel’s fibers also fell. The scientists then used a computer to mathematically analyze this relationship. Their findings suggest that at a pH of 7.5, a mussel’s ability to hang onto rocks is only about 40 percent as good as at a pH of 8!
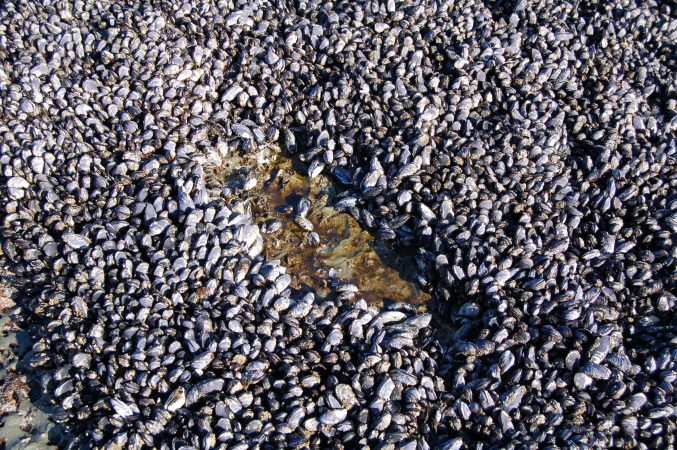
Living in warmer and more acidic waters means that seashore mussels will have a tougher time clinging to rocks. (And that’s not even counting the fact that climate change could make waves stronger and more frequent.) If mussels can’t cling to rocks as effectively, then large patches of them could wash away. That would bring big changes to coastal ecosystems. For instance, it could open large patches of coastal real estate to invasion by seaweed or other invasive species.
Mussel farmers could also feel the pinch. These people grow the bivalves on ropes dangling in the water. The new findings suggest they’ll probably have to use longer ropes so the mussels can live in cooler, deeper waters. And the requirement for longer ropes means it will raise the costs of growing mussels. But the added expense might be worth it: Mussels are big business. The global sales of this yummy shellfish now exceed $1.5 billion.
Power Words
acidification A process that lowers the pH of a solution, making it more acidic. When carbon dioxide dissolves in water, it triggers chemical reactions that create carbonic acid.
biomedical engineer Someone who uses science and math to find solutions to problems in biology and medicine. For example, they might create medical devices such as artificial knees.
bivalve An invertebrate that lives in a two-part, hinged shell. Most bivalves filter their food from the waters that slosh past them. Bivalves include clams, oysters, scallops and mussels.
fetus A stage in the prenatal development of mammals and live-bearing reptiles. That stage is lengthy and is typically the last stage before birth.
fossil fuels Any fuel (such as coal, oil or natural gas) that has developed in the Earth over millions of years from the decayed remains of bacteria, plants or animals.
insoluble Incapable of being dissolved into a fluid or gas. Salt and sugar can dissolve in water, for example, but some other substances, including some of those with large molecules such as proteins, do not.
invertebrate A creature without a backbone. About 97 percent of the world’s species are invertebrates. They include insects, worms, snails, octopuses and starfish, to name just a few.
materials scientist A scientist who studies how the atomic and molecular structure of a material is related to its overall properties. Materials scientists can design new materials or analyze existing ones. Their analyses of a material’s overall properties (such as density, strength and melting point) can help engineers and other researchers select materials that are appropriate for a particular new use.
marine biologist A scientist who studies creatures that live in ocean water, from bacteria and shellfish to kelp and whales.
molecular biologist A scientist who studies the processes that take place inside an organism’s cell.
pH A measure of a solution’s acidity. A pH of 7 is perfectly neutral. Acids have a pH lower than 7; the farther from 7, the stronger the acid. Alkaline solutions, called bases, have a pH higher than 7; again, the farther above 7, the stronger the base.