Cool Jobs: Puzzling over proteins to study life and death
Three research groups are discovering how tiny machines power cells, people and other animals
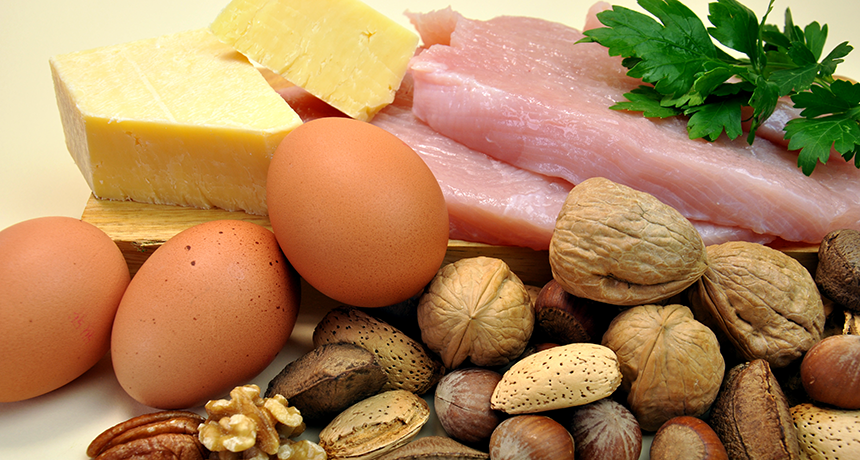
Most people think of fibrous and muscle-rich foods when they hear the word protein. In fact, every cell of the body makes proteins, which will carry out all of its many functions. Scientists are focusing on proteins — in cells and foods and fossils — to better understand how the body functions.
MillefloreImages/iStockphoto
By Bryn Nelson
Mary Schweitzer and her research team were studying the fossil of an 80-million-year-old duck-billed dinosaur. Suddenly, they noticed something odd. When they examined the dinosaur’s leg bone, they found ancient fragments of a protein called collagen (KAHL-eh-jen). This common protein is found in the cartilage, bones, skin and muscles of many animals, including humans. Schweitzer’s team didn’t expect to find any trace of a protein in something so old.
Schweitzer is a dinosaur expert at North Carolina State University in Raleigh. She and her team want to understand more about how dinosaurs lived and died. But they’re not focusing only on the shape and size of fossils. Instead, they’re digging into bones to reveal what the human eye can’t see. This team is studying the chemistry of those ancient bones.
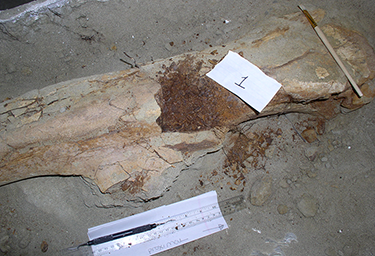
This discovery and others have taught them an important lesson. “Fossils are not rocks,” Schweitzer says. “They actually have pieces and parts of the once-living animals within them.” Some fossils may contain soft and stretchy tissue, blood vessels and cells, she has found. Some can even hold onto chemicals like proteins, which scientists thought were too fragile to survive millions of years.
How can such biological materials last that long? This is just one of many questions being asked of proteins, tiny chemical machines within our cells.
DNA provides each cell with an instruction book on how to make these bitty widgets. The machines a cell builds then do all the work to help it survive. Proteins carry in crucial supplies and take out the trash. They send important messages. They even fight off invaders.
Studying proteins gives scientists a better idea of how cells are supposed to work and what happens when they malfunction.
Schweitzer is among researchers taking a closer look at proteins’ many roles. A children’s doctor in St. Louis, Mo., is part of another team studying how protein-rich peanut butter could work like medicine to help kids in Africa. And a scientist in Seattle, Wash., is using glowing jellyfish proteins to light up — and label — parts of living cells so that she can see how they work.
Block by block
Cells make proteins by piecing together basic chemical building blocks known as amino (Ah-MEE-no) acids. Our cells choose from a standard kit of only 20 different amino acids. But cells can string them together in countless ways. The result is a remarkably diverse catalog of proteins.
So far, researchers have found the basic instructions — or genes — for about 21,000 human proteins. Including possible variations, though, the total number of different types could be as high as 250,000 to one million. Some are only needed for a short time. Cells can then recycle their amino acid parts to build new proteins. Others, such as collagen proteins, provide tissues such as bone and muscle with solid supports that are built to last.
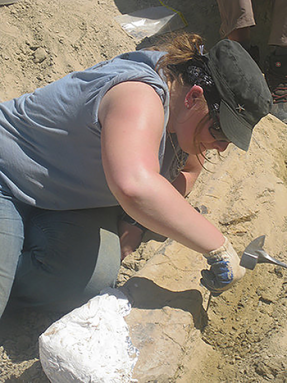
Collagen’s strength comes from its structure. The amino acids in collagen I form a complicated braid known as a triple helix. This sturdy frame can link up with its neighbors to make a scaffold. Researchers think these connected supports keep collagen I from falling apart.
Finding collagen in fossils was a surprise. Recognizing its staying power might now prove quite useful. Schweitzer and Elena Schroeter have begun using proteins as clues to figure out how dinosaurs lived, grew and evolved. Schroeter is a postdoctoral researcher in Schweitzer’s lab. (She has her doctoral degree but is continuing to study before starting her own lab.)
Until recently, to study the dinosaur family tree paleontologists mostly relied on the shapes of related animals’ bones. The idea, here, is that an animal’s skeleton will be most similar to that of its closest relatives. “As you get more distantly related, the skeleton is [increasingly] different,” Schroeter explains.
But dinosaur fossils are rare. Researchers often don’t have complete dinosaur skeletons. Bone comparisons only work if researchers can match up the same bones from different animals. For instance, Schroeter explains, you can’t compare an arm bone from one dinosaur to a tailbone from a second.
Biologists often compare the DNA of living animals to find out how closely related they are. But DNA is fragile and hard to collect from fossils. That’s why proteins could help. Collagen, for example, is less fragile than DNA. That should make it easier to study. And because DNA provides the template for making collagen, the protein can still point out differences among animals’ instruction books.
“The collagen in my arm bone and the collagen in my leg bone is the same,” Schroeter says. Slight differences in the protein between species might reveal how closely related the two animals were — and how they evolved over time.
Protein power
To collect dinosaur protein, Schroeter grinds up a fossilized bone. Then she adds the powder to chemicals in a liquid. These chemicals help separate protein pieces from mineral bits. A machine called a mass spectrometer can help identify the protein bits. This machine acts like a sensitive scale. It measures the mass of every protein fragment in the mixture. A computer program then matches those fragments to ones from known proteins. Like trying to fit puzzle pieces together, it determines which pieces are present. It also predicts how they would match up to form the original protein.
Someday, the methods used to identify dinosaur proteins might even help in the hunt for life on Mars. In both cases, Schweitzer explains, researchers would likely look for very old and tiny pieces of some type of molecule. If found and properly identified, molecules (such as proteins) might offer signs of long-ago life.
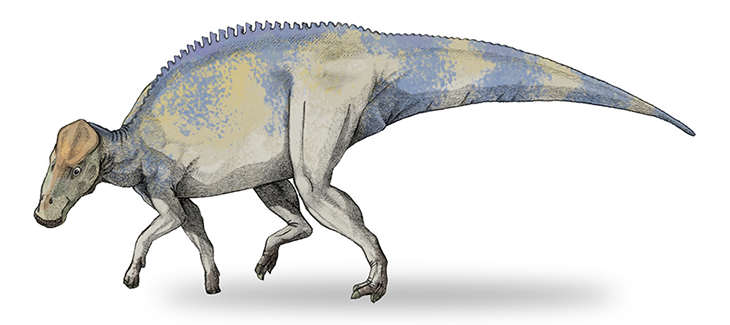
So far, Schweitzer and Schroeter have pieced together more than 10 percent of the collagen I protein from the duck-billed dinosaurs. That’s enough to place this animal between crocodiles and birds on the family tree of animals. More complete proteins might point out new links to other dinosaurs as well.
To fill in the family tree, Schweitzer and Schroeter are looking for collagen and other proteins in more fossils from duck-billed dinosaurs, from Tyrannosaurus rex and from other species.
Beyond showing how dinosaurs are related to animals that live today, proteins might unlock other secrets of these extinct animals. Imagine if researchers could identify proteins and other contents in a dinosaur’s stomach. If so, Schweitzer says, we might discover how certain dinosaurs became giants — like the sauropods. These plant-eaters, known for their long necks and tails, had unusually small mouths. Yet they could eat enough to grow from an egg to adults weighing more than 80 tons. How could they reach nearly half a football field in length?
Maybe ancient plants were more nutritious. Maybe the dinosaurs could digest plants more efficiently. In fact, learning what helped sauropods grow so big might help researchers identify old proteins that could prove useful even today.
“Dinosaurs had a lot of stuff figured out that we mammals haven’t achieved nearly so well,” Schweitzer says. Proteins and other molecules, she says, may “hold the key.”
Protein power
Protein isn’t just important for studying bones. It’s a vital component of our diet. It’s found in food such as eggs, meat and milk. When you eat protein, your body dissolves it into its amino-acid building blocks. Those blocks can then be recycled to build new proteins to make muscles. (That’s why bodybuilders eat so much high-protein food.) During childhood, kids also need plenty of protein for the construction projects taking place throughout their bodies.
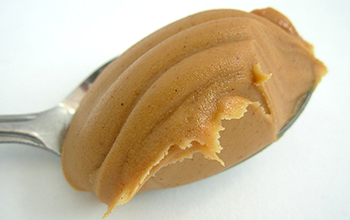
If children don’t get enough to eat — or enough protein overall — their health will suffer. But proteins in some foods, such as peanut butter, can pack a real punch.
Mark Manary is a pediatrician at Washington University in St. Louis. He’s always been driven to try and make the world a better place. After medical school, he worked at a hospital in the southeast African country of Malawi. There, he confronted a big problem. “Kids were sick because they didn’t get enough to eat,” he recalls. They were suffering from a life-threatening condition called severe acute malnutrition.
And these kids were not alone. The United Nations International Children’s Emergency Fund, or UNICEF, estimates that worldwide 16 million children under age 5 suffer from this condition. These kids are nine times as likely to die as are children who get enough healthy food.
“One of the most important capacities [our bodies] have is the ability to fight infection,” Manary explains. When malnourished, kids’ defenses begin to fall apart. “Your body needs these building blocks. It needs fresh vitamins and minerals and protein and everything to rebuild and restore itself every day,” he says. “When it doesn’t get it, it starts wasting away.”
But it was difficult to provide malnourished kids with the right mix of protein-rich foods. In the hospital, Manary tried feeding them milk and other nutritious foods. But fewer than half of the children got better.
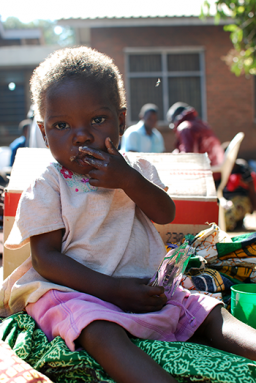
In fact, keeping kids in the hospital for a long time often made them worse. They often picked up diseases from other kids, notes Meghan Callaghan. She helps coordinate Manary’s research. In Malawi, parents sometimes took their kids home before they were well. At home, such families often struggled to provide the extra food their children might need.
The peanut butter solution
Nearly 20 years ago, Manary tested a special food rich in protein and energy (fat) that African families could feed these kids. He wanted something that wouldn’t spoil in warm weather and wouldn’t need to be cooked. It had to contain little water so that germs would not grow well in it.
“I’m an American,” he notes. “What’s in your cupboard that meets those criteria? Peanut butter!”
After Manary’s aha moment, he and his colleagues tested this idea on thousands of children who were being sent home from the hospital. He gave their mothers a paste made from peanut butter, dried milk, sugar, vegetable oil, vitamins and minerals. The bonus ingredients helped boost the paste’s nutritional value. Africans commonly eat ground peanuts, so the kids had no problem with this “medicine.” Manary told the parents to bring their kids back for regular check-ups. Each time, he checked how they were doing.
In one study with some 1,200 children, Manary compared rates of recovery in kids who had been hospitalized with severe acute malnutrition. Some were sent home with his peanut-butter treatment. Others stayed in the hospital, receiving typical care. And the kids treated at home were twice as likely to recover.
Additional studies confirmed the peanut-butter therapy’s success in helping kids with severe malnutrition. Many of these kids had a type of disease called kwashiorkor (QWASH-ee-OR-kor). It’s a life-threatening condition caused by a severe shortage of protein.
Based on their success, Manary and his colleagues started a nonprofit group in 2004. They wanted to help spread their treatment plan to even more communities. Their Project Peanut Butter opened food factories in three African nations: Malawi, Sierra Leone and Ghana.
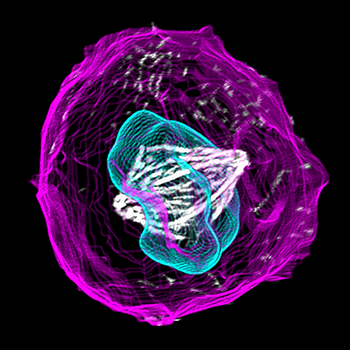
A cell’s “Google map”
To find out what proteins actually do in the body, other scientists are creating what they’re calling a “Google map of the cell.” Ru Gunawardane works in Seattle. There, she directs a stem-cell and gene-editing program at the Allen Institute for Cell Science. Her team is studying how proteins help our cells divide, move and communicate. These scientists also are making their maps of living cells and other information available to researchers elsewhere around the world.
Gunawardane’s research is aiding in the discovery of how parts of a cell all work together. She often tries to look at many cells at once to see how they and their proteins interact. Some proteins, for example, may help cells stick together while they exchange messages. “Cells love to be in a group. They have their own cliques,” she says.
Proteins have another important job: managing how a cell divides. Diseases like cancer occur when cells no longer divide as they should. Instead, their “growth has gone crazy,” Gunawardane says. To better understand this, she is studying proteins in a cell’s nucleus.
She also is examining proteins that act as structural supports. These sturdy proteins keep a cell from collapsing and dying. Still other cell proteins have jobs that aren’t entirely clear. Based on advice from other scientists and research, Gunawardane’s team is focusing on about 20 different proteins.
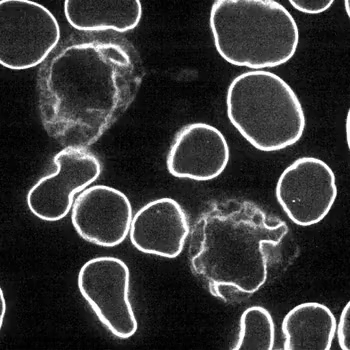
One challenge is that proteins are too small for the eye to see. To figure out how proteins work — or fail — in real life, researchers want to see how they move inside living cells. To follow their action, researchers add glowing tags to mark the proteins they’d like to track.
Each bright tag is a green fluorescent protein that jellyfish make (and that scientists often use now in their research). Gunawardane and her colleagues can attach this glowing beacon to a human protein, making it easy to spot. To look at more than one protein, the researchers sometimes tag other cells with a red fluorescent protein.
When scientists then look through a powerful microscope, they now can see where each glowing protein is. Gunawardane compares the method to using a tracking device to see where your dog goes. In her case, she can watch what a lit-up protein does as its cell divides, moves or “chats” with its neighbors.
Gunawardane is particularly interested in what happens as human cells grow and develop. Proteins assist in a complex process that transforms a group of cells into tissues, organs and even a whole organism.
“We’re working with stem cells, which have this amazing ability to become any cell type in the body,” she says. “We really want to know how the cell decides to stay a stem cell or become a completely different cell type.” In other words, who’s the boss that tells the cell to become a heart cell, lung cell or brain cell?
Peeking inside living cells has already revealed surprises. One case taught Gunawardane’s team about cellular recycling. They tagged a protein attached to a membrane that surrounds — like an envelope — all the parts of a cell’s nucleus.
“I always thought that the nuclear envelope completely goes away when the cell is dividing,” she says. Instead, her team saw that some of the envelopes stays intact. That way, both new cells can reuse the leftovers to help stitch together membranes to cover their new nuclei.
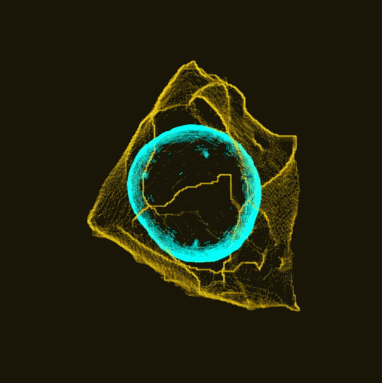
If scientists know which proteins help make key decisions, Gunawardane says, they can ask which ones are misbehaving in sick cells. That knowledge could lead to new drugs that lessen or prevent errors by the sick cells.
“I was always curious about what goes wrong in disease,” she says. Even though mistakes in our genes may trigger the damage, she realized, our proteins are what do the harm. Whether they’re missing, damaged or excessive, proteins can be the difference between sickness and health.
Proteins, then, could help shape the future of medicine. Along with helping kids in the present and revealing dinosaur secrets from the past, these mini-machines are proving their mighty power.