Phoning in earthquakes
Networks of mobile devices could detect tremors and allow early warning of big shakes to come
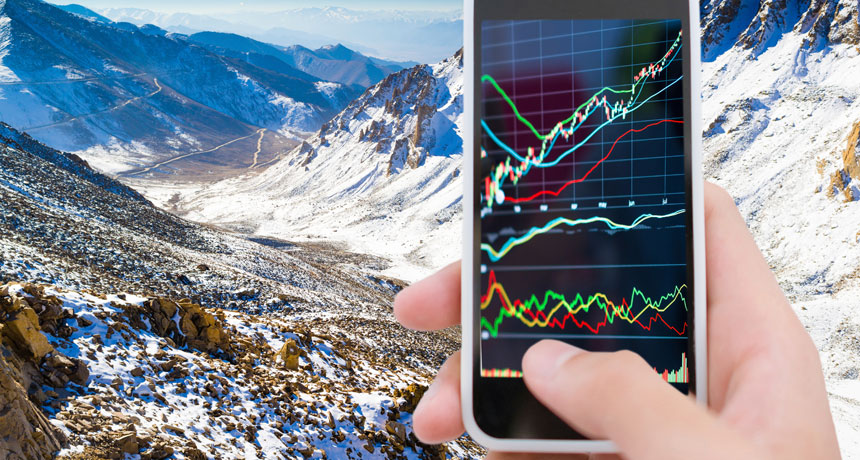
After downloading an app onto your cell phone, the device can use motion sensors inside it to detect quakes. The energy waves of those ground shakes can be graphed, resembling at some level the stock trends depicted on this smart phone. The app can then automatically send details of the time, place and intensity of those tremors to scientists. Thiscrowdsourcing of data may soon give researchers a low-cost way to map quakes across a broad region.
Teradat Santivivut/ iStockphoto
At 3:20 a.m. on August 24, 2014 — not quite a year ago — a strong earthquake rocked the northern California town of Napa. It sparked fires. Historic buildings crumbled. And more than 200 people were hurt. This region had not experienced ground shaking that powerful in nearly a quarter-century.
Less than one minute later, about 38 kilometers (24 miles) south of the Napa quake’s underground origin, or epicenter, the quake’s waves reached the city of Berkeley. There, the waves swayed the apartment building where Qingkai Kong lay asleep.
Kong studies earthquake detection systems at the University of California, Berkeley. For this graduate student, the Napa quake was real — and a test. Bleary-eyed, Kong stumbled out of bed and glanced at an instrument on his desk. He saw the familiar flurry of jagged lines that signal an earthquake had occurred.
For many years, the only instruments that could provide that kind of information cost thousands of dollars. This instrument was different: It was Kong’s Android smartphone.
Kong’s phone was running MyShake. This software application — or app — uses the inexpensive sensors in mobile devices to record ground movements. The squiggly waveforms of the Napa quake captured on his phone looked quite similar to those recorded by a nearby scientific sensor, Kong told Science News for Students.
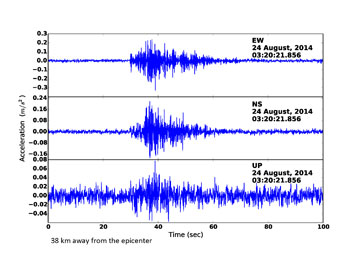
Together, these citizen science projects are helping researchers create networks of instruments to measure earthquakes quickly and reliably. The efforts also are building awareness of the dangers that quakes pose and how to prepare for them. Eventually, these networks could underpin early-warning systems that can save lives.
In San Francisco, Calif., last December, Kong and others described some of these citizen networks. They also reported new data from these networks there at the fall meeting of the American Geophysical Union, or AGU.
Earthquake basics
Earthquakes are common: Several million shake our planet each year. Recently, scientists have detected even more vibrations in areas where groundwater is pumped to the surface or where carbon dioxide is injected belowground.
Most earthquakes are too small for people to feel. Such tiny quakes register less than magnitude 3.0. By comparison, the south Napa earthquake was a magnitude 6. Such scores rate the intensity of ground motion triggered by a quake.
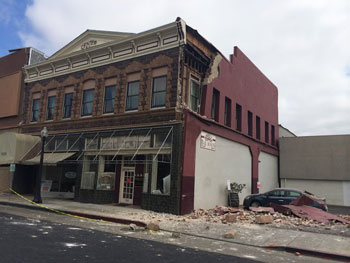
Given their size and big price tag, quake mappers can’t plunk these seismographs just anywhere, explains Jennifer Strauss. She works at UC Berkeley’s Seismological Laboratory. There she helps connect its research to the needs of people and companies. Explains Strauss: “You can’t put one on a hillside where birds will run into it or disturb it. Or next to a highway where every truck going over a bump is going to register a signal.” Scientists instead must choose carefully where they install these instruments.
Fortunately, seismic sensors are shrinking in size and cost. Since the sensors send data over the Internet, operating costs are falling too.
As a result, researchers can afford to place more instruments in more places. These higher-density networks can better pinpoint where earthquakes start. The devices also can warn of smaller tremors, called aftershocks, which often follow a major quake.
Crowd-sourced science
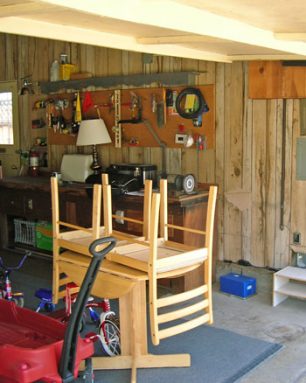
Since 2009, USGS has invited volunteers to help expand its seismic network. The agency’s scientists have placed about 500 low-cost, car-battery-sized seismographs in and near the places where people live, work and study. These little blue boxes detect ground movements in earthquake-prone urban areas throughout the United States. Most have been placed in and around Seattle, Los Angeles and San Francisco.
These “NetQuakes” sensors are not as sensitive as the bigger professional instruments. Still, the low-cost devices can detect quakes of magnitude 1 or 2. (For each increase in magnitude, an earthquake produces 10 times more ground motion. It also releases about 32 times more energy.)
And still smaller and cheaper quake sensors are becoming available. Some attach to personal computers using a USB port. After a volunteer connects the box and downloads a web application, the sensor is “ready to go,” explains Monica Kohler. She is an engineer at the California Institute of Technology in Pasadena. “It records shaking from that site and sends info continuously to us. And the box is very inexpensive: It costs $300.”
At the December AGU meeting, Kohler and her coworkers presented their latest efforts in building the Community Seismic Network. Since 2011, this team has placed low-cost sensors at some 400 homes and offices in or near Los Angeles. These sensors can only detect quakes that are magnitude 3 or higher. That makes them less sensitive than even the USGS’s NetQuakes devices.
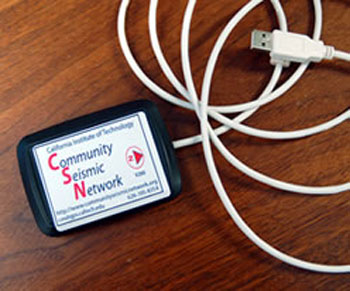
Data from seismometers go into producing “shake maps.” Researchers analyze these maps to determine which areas experienced earthquake vibrations. The maps can show where the ground is shaking with an accuracy of 10 kilometers to 20 kilometers (6 miles to 12 miles). However, “if you want to detect shaking on a block-by-block scale, you need more sensors placed more densely,” Kohler notes.
Because the newest seismic sensors are so cheap, they are allowing researchers to expand the quake-mapping network. Potentially this network could include thousands — even tens of thousands — of stations, Kohler says.
Laptops and phones
Cheaper sensors can spawn denser networks of seismic instruments. One example is the Quake-Catcher Network (QCN). It has recruited roughly 3,400 volunteers around the globe.
Some volunteers host quake sensors that resemble the finger-sized USB flash drives commonly used to store and transfer computer files. Others instead collect seismic data using motion sensors called accelerometers. Most laptops, tablets and smartphones hit the stores already loaded with these sensors.
(Story continues below image)
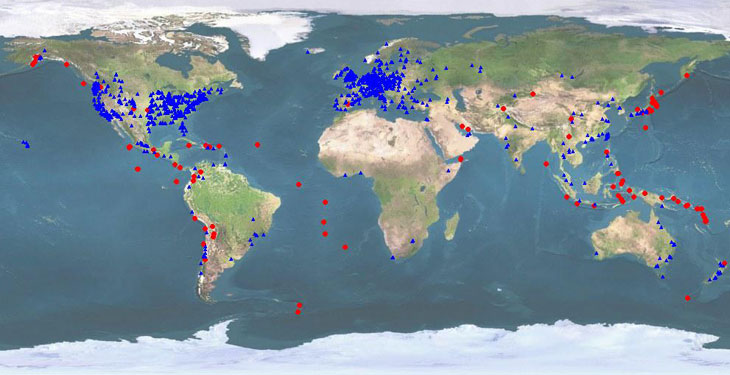
As their name implies, accelerometers detect motion due to acceleration forces. Acceleration is the rate at which the speed or direction of something changes over time. In smartphones, it’s the accelerometer that senses when you rotate a phone — and then signals the display to rotate its image to match.
The accelerometer in a mobile device is sensitive enough to detect a magnitude 3 to 3.5 earthquake whose epicenter is a few kilometers (miles) away. The accelerometer in a desktop sensor can record quakes as small as magnitude 2.5. If people have downloaded the QCN app, their devices will send a report to scientists of any ground movement that the sensors in their phones or computers have picked up. Researchers can then pool and analyze all of the data that these devices capture.
“With these additional sensors, we could potentially detect an earthquake more quickly,” says Elizabeth Cochran. She is a geophysicist at the USGS Earthquake Science Center in Pasadena. “With more data, you can get faster and more reliable estimates of earthquake location and magnitude.”
Within towns and buildings
Alaska is an earthquake hotspot. There, Quake-Catcher sensors already have been put to the test. In 2014, these devices were installed at 24 schools in Anchorage, Alaska’s largest city. Their installation coincided with the 50th anniversary of the 1964 “Good Friday earthquake.” This magnitude-9.2 whopper shook the ground for nearly 5 minutes. It was the second-largest earthquake ever recorded.
In September 2014, a magnitude-6.2 quake struck 130 kilometers (81 miles) northwest of downtown Anchorage. “In some areas, there was just rattling,” recalls Kathryn Kurtz. “In others, things were falling off shelves.” Kurtz coordinates math and science curriculum for the Anchorage schools fitted with the Quake-Catcher sensors. Several of these instruments detected the shaking.
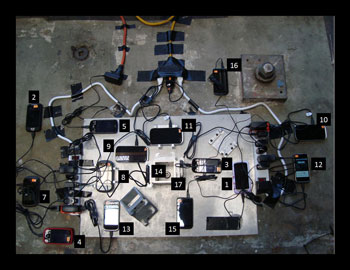
Buildings and other structures face a particular risk of damage if built on a type of clay formed by ancient glaciers. Known as quick clay, this sediment liquefies when the ground shakes. On the other hand, buildings on bedrock hardly budge. “The cool thing about the seismographs is they gave us a sense of where in town the quakes are felt more,” Kurtz says.
In southern California, smaller earthquakes can cause fractures in high-rise buildings. Some of the cracks likely will be “too small to see with the naked eye,” Kohler notes. “Yet they’ve probably occurred in many buildings and left them in a weakened state.”
Kohler’s team at Caltech has installed sensors in about 10 high-rise buildings in the Los Angeles area. One building has sensors on each of its 52 floors. “It’s amazing the pictures we’re getting,” Kohler says. “We can see how the building moves on an hourly basis.” By watching how buildings sway in the wind — or in response to an earthquake — the researchers can scout for signs that it may need strengthening. Such fixups are called retrofitting. And they can make buildings more quake-resistant.
Shimmy sim city
Luckily, destructive earthquakes are rare. Strong shaking only happens occasionally — and at times and in locations no one can predict. But that also makes it difficult for earthquake researchers who need to test their sensors.
One solution is to use a “shake table.” Some are able to hold rooms or small buildings. Engineers program these machines to jiggle and jerk, mimicking the ground motions that occur during an earthquake. “You input seismic waves to simulate an earthquake and put smartphones on the table to see what they record,” explains Kong.
SHAKE IT This roughly 3-minute clip shows a building on a “shake table” and how it responds to a simulated earthquake. Discovery News |
Recently, a different team of researchers conducted an earthquake simulation. This experiment put a virtual network of quake-sensing smartphones to the test. The results suggest that such a network could warn some people in San Francisco up to 3 seconds before they would encounter the effects of a magnitude-7 quake across the San Francisco Bay, about 28 kilometers (17 miles) to the east. Details appeared April 10 in the journal Science Advances.
Early warnings
Earthquakes can’t be predicted. Still, early-warning systems can let people know a quake has begun. Depending on their distance from a quake’s epicenter, sensors in Japan and Mexico already can provide warnings to some people there seconds ahead of any shaking. That might not seem like much. Still, it can be enough time for people to take cover — or for automated equipment to take action. Elevators might automatically stop at the nearest floor. Firehouse doors could swing open to allow trucks to exit.
These warnings are possible because digital communication moves at near the speed of light. By contrast, seismic waves travel through the ground more slowly, at only the speed of sound. It also helps that there’s a lag time between the arrival of an earthquake’s primary waves (p-waves) and secondary waves (s-waves). P-waves are speedier. They’re the first to signal an earthquake. But the follow-up s-waves are stronger. That means they’re also more destructive.
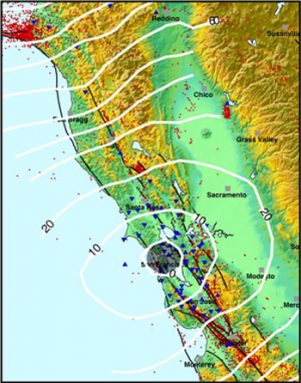
The United States does not yet have an earthquake warning system in place. In December, Congress awarded $5 million to USGS for a West Coast earthquake early-warning system called ShakeAlert. Firefighters, police and other emergency responders, along with some private companies, have been testing the system since 2012. However, before it can be deployed further, the system needs more testing — and another $33 million, USGS scientists say.
The West Coast ShakeAlert system is now undergoing testing with about 625 sensors. Eventually, researchers hope to expand the system. They’ll add smartphones and inexpensive low-cost quake sensors to the costlier network of seismographs that will form the backbone of this early-warning system. Doing so should greatly expand the system’s reach. The San Francisco Bay Area alone has millions of smartphone users. Points out Strauss, “If we could harness people’s smartphones that they have with them every day, all day, then we’d have a vast seismic network easily available.”
Power Words
(for more about Power Words, click here)
acceleration The rate at which the speed or direction of something changes over time.
accelerometer An instrument for measuring vibrations or a change in the rate of movement. These sensors typically can measure movement changes in all three dimensions (front-to-back, side-to-side and up-and-down).
aftershock One or more smaller earthquakes that often follow a major earthquake.
bedrock The thick, solid rock layer than underlies the soil and other broken, rocky materials on Earth’s surface.
carbon dioxide A colorless, odorless gas produced by all animals when the oxygen they inhale reacts with the carbon-rich foods that they’ve eaten. Carbon dioxide also is released when organic matter (including fossil fuels like oil or gas) is burned. Carbon dioxide acts as a greenhouse gas, trapping heat in Earth’s atmosphere. Plants convert carbon dioxide into oxygen during photosynthesis, the process they use to make their own food.
concentric A series of circles or rings that have a common center point.
citizen science Scientific research in which the public — people of all ages and abilities — participate. The data that these citizen “scientists” collect helps to advance research. Letting the public participate means that scientists can get data from many more people and places than would be available if they were working alone.
clay Fine-grained particles of soil that stick together and can be molded when wet. When fired under intense heat, clay can become hard and brittle. That’s why it’s used to fashion pottery and bricks.
crowdsourcing A term coined in 2005 for the collection of data from a large community of volunteers — often over the Internet. For instance, those volunteers may collect information intentionally (such as data on cloud cover, the appearance of a particular butterfly or a recording of the call of a certain bird), then send the data to some researcher. Alternatively, an app downloaded on someone’s phone might collect light, vibrations or some other information periodically — and automatically — and then relay it over the Internet to researchers.
curriculum (plural: curricula) The official classroom materials (often readings) used to lead students through a course of study on a particular topic.
earthquake A sudden and sometimes violent shaking of the ground, sometimes causing great destruction, as a result of movements within Earth’s crust or of volcanic action.
epicenter The underground location along a fault where an earthquake starts.
flash drive A type of small data-storage device (typically smaller than a pack of chewing gum) that can receive or transmit digital data through a USB port.
force Some outside influence that can change the motion of a body, hold bodies close to one another, or produce motion or stress in a stationary body.
glacier A slow-moving river of ice hundreds or thousands of meters deep. Glaciers are found in mountain valleys and also form parts of ice sheets.
geophysics The study of matter and energy on Earth and how they interact.
global positioning system Best known by its acronym GPS, this system uses a device to calculate the position of individuals or things (in terms of latitude, longitude and elevation — or altitude) from any place on the ground or in the air. The device does this by comparing how long it takes signals from different satellites to reach it.
graduate student Someone working toward an advanced degree — typically a Master’s degree of PhD — by taking classes and performing research. This work is done after the student has already graduated from college (usually with a four-year degree).
groundwater Water that is held underground in the soil or in pores and crevices in rock.
liquefy (in geology) A term for the movement of soil particles during an earthquake that keeps them from holding firm and serving as a solid foundation for buildings, roads, bridge footings and other structures.
magnitude (in geology) A number used to describe the relative size of an earthquake. It runs from 1 to more than 8 and is calculated by the peak ground motion as recorded by seismographs. There are several magnitude scales. One of the more commonly used ones today is known as the moment magnitude. It’s based on the size of a fault (crack in Earth’s crust), how much the fault slips (moves) during a quake, and the energy force that was required to permit that movement. For each increase in magnitude, an earthquake produces 10 times more ground motion, and releases about 32 times more energy. For perspective, a magnitude 8 quake can release energy equivalent to detonating 6 million tons of TNT.
network A group of interconnected people or things.
seismic wave A wave in the ground produced by an earthquake or other means.
seismometer (also known as a seismograph) An instrument that detects and measures tremors (known as seismic waves) as they pass through Earth.
seismology The science concerned with earthquakes and related phenomena. People who work in this field are known as seismologists.
sensor A device that picks up information on physical or chemical conditions — such as temperature, barometric pressure, salinity, humidity, pH, light intensity or radiation — and stores or broadcasts that information. Scientists and engineers often rely on sensors to inform them of conditions that may change over time or that exist far from where a researcher can measure them directly.
simulate To deceive in some way by imitating the form or function of something. A simulated dietary fat, for instance, may deceive the mouth that it has tasted a real fat because it has the same feel on the tongue — without having any calories. A simulated sense of touch may fool the brain into thinking a finger has touched something even though a hand may no longer exists and has been replaced by a synthetic limb. (in computing) To try and imitate the conditions, functions or appearance of something. Computer programs that do this are referred to as simulations.
smartphone A cell (or mobile) phone that can perform a host of functions, including search for information on the Internet.
software The mathematical instructions that direct a computer’s hardware, including its processor, to perform certain operations.
universal serial bus (abbreviated USB) A type of hardware technology used to connect some peripheral device — such as a mouse, keyboard or thumb drive — to a computer, smartphone, digital camera or other electronic system.
urban Of or related to cities, especially densely populated ones or regions where lots of traffic and industrial activity occurs. The development or buildup of urban areas is a phenomenon known as urbanization.
USB port The opening on a computer or other digital device into which a USB connector can be inserted.
U.S. Geological Survey, or USGS This is the largest nonmilitary U.S. agency charged with mapping water, Earth and biological resources. It collects information to help monitor the health of ecosystems, natural resources and natural hazards. It also studies the impacts of climate and land-use changes. A part of the U.S. Department of the Interior, USGS is headquartered in Reston, Va.
vibrate To rhythmically shake or to move continuously and rapidly back and forth.
virtual Being almost like something. An object or concept that is virtually real would be almost true or real — but not quite. The term often is used to refer to something that has been modeled — by or accomplished by — a computer using numbers, not by using real-world parts. So a virtual motor would be one that could be seen on a computer screen and tested by computer programming (but it wouldn’t be a three-dimensional device made from metal).
wave A disturbance or variation that travels through space and matter in a regular, oscillating fashion.
wifi (also Wi-Fi) A wireless technology that networks various electronic devices (such as cell phones and laptop computers); it allows them to share the same modem for Internet connections by using radio waves.