Sea creatures’ fishy scent protects them from deep-sea high pressures
Physicists worked out how a chemical called TMAO helps fish endure crushing undersea pressures
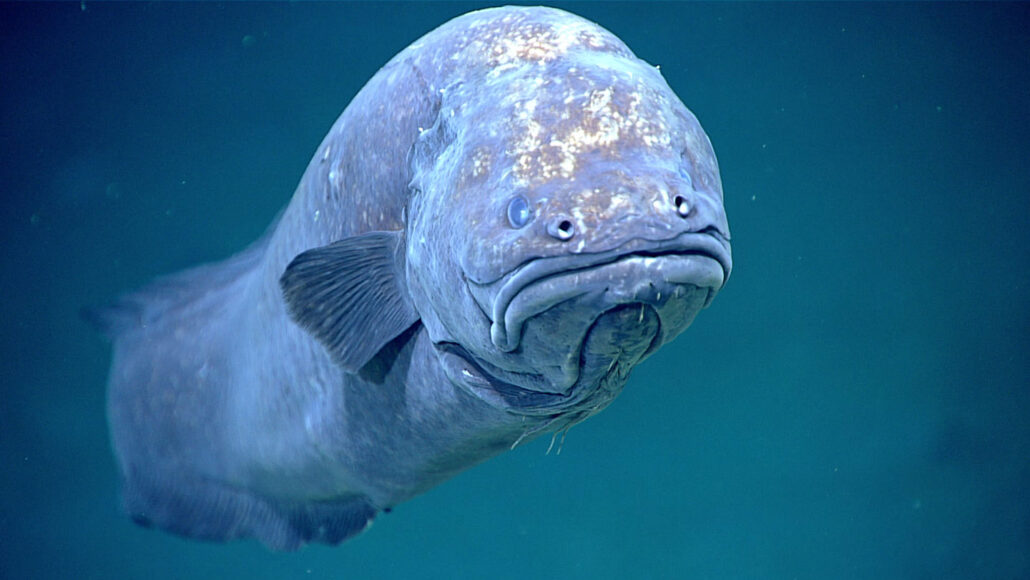
This particularly grumpy-looking ophidiiform cusk eel had its picture snapped at a depth of 1,585 meters (5,200 feet). Its tissues hold a crucial chemical that enables it and other deep-sea fish to endure extreme underwater pressures.
NOAA Office of Ocean Exploration and Research/Gulf of Mexico 2017
The biggest obstacle to living at our ocean’s greatest depths isn’t the cold or perpetual darkness. It’s the intense pressure that comes from living under a column of seawater many kilometers (miles) deep. Yet some seemingly fragile, non-armored fish live there comfortably. Scientists have seen hints that as the depth of the watery ecosystem increases, one chemical in a fish’s body increases. But how it might help creatures withstand what should be bone-crushing pressures remained a mystery. Until now.
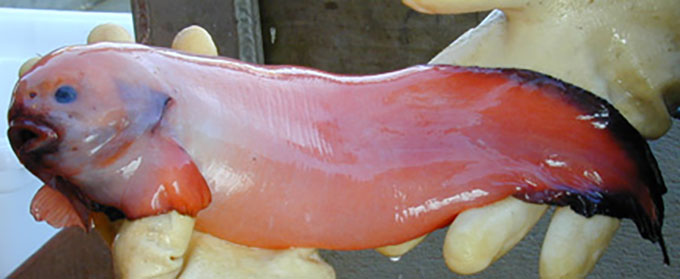
The new discovery teaches us how life “has adapted to extreme environmental conditions,” says Lorna Dougan. She’s a physicist at the University of Leeds in England. Her team published its new findings in the September 2022 Communications Chemistry.
Learning how this chemical works might also help other research fields where life’s molecules must withstand pressure. Biomedicine is one example. The food industry is another.
The chemical is known as TMAO. That’s short for trimethylamine (Try-METH-ul-uh-meen) N-oxide. You probably haven’t heard of it, says Paul Yancey — a marine biologist at Whitman College in Walla Walla, Wash. But “everyone has smelled it that’s ever been to a fish market.” TMAO is what gives aquatic species their fishy scent.
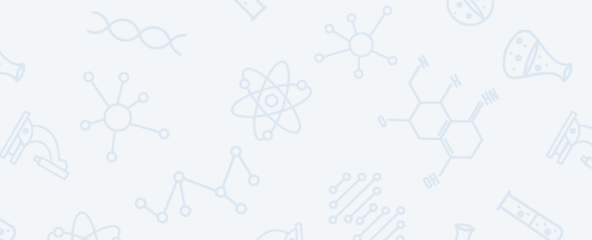
Educators and Parents, Sign Up for The Cheat Sheet
Weekly updates to help you use Science News Explores in the learning environment
Thank you for signing up!
There was a problem signing you up.
In 1998, Yancey first discovered why fish have this smelly chemical. “We were on a deep-sea expedition,” he recalls. His team was capturing fish at various depths. Afterward, they measured TMAO levels in the animals’ muscles. Deep-sea species had more TMAO than shallow species.
Even more interesting, that relationship was linear. Like pressure, it changed at a fairly constant rate with depth. Lots of environmental features change with depth, Yancey notes. But only pressure changes in this linear way. So that was a nice link to the TMAO data. His team published that study in the Journal of Experimental Zoology. Follow-up studies by others now confirm what had been Yancey’s hunch — that this stinky chemical is the fishes’ adaptation to high pressure.
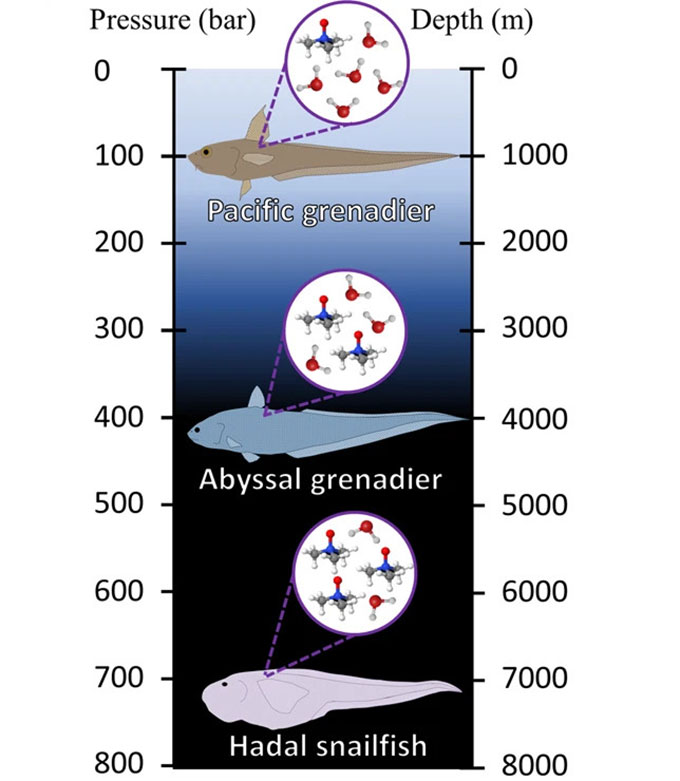
“I’m not a physical chemist,” Yancey says, “so I couldn’t analyze the mechanism.” But in the new study, the British team has picked up where his left off. It used physics to unlock the secret workings of this molecule.
Under pressure, even water gets wacky
Water molecules normally stick together like little magnets. They form a tetrahedral (pyramid-like) structure. That gives water many of its special properties. For example, it explains how a water strider can skitter across a pond surface without sinking.
But extreme pressure squashes this network of water molecules. That’s especially true in the oceans’ deep trenches. It’s known as the hadal zone (named for the Greek god Hades who ruled the underworld). There, the pressure is “about the equivalent of an elephant standing on top of your thumb,” says Mackenzie Gerringer. She’s a marine biologist at the State University of New York (SUNY) in Geneseo. And that pressure doesn’t just press down. It pushes in from all sides, too.
“The weight of the water pushes water molecules into proteins and distorts them,” Yancey explains. Proteins have complex 3-D shapes. And if that shape gets warped, those proteins “can’t work very well.” That would cause problems because proteins, he notes, are the “universal machinery of life.” And the British team has now shown how TMAO can protect proteins under pressure.
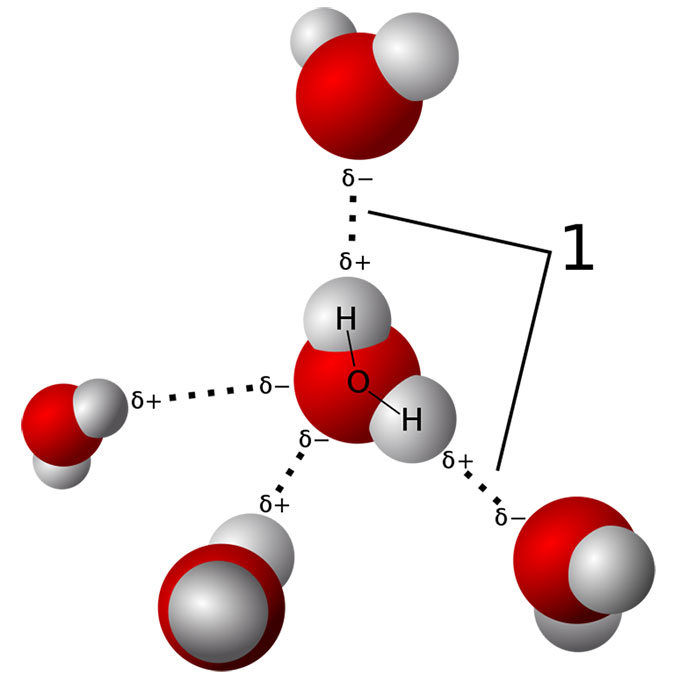
Dougan and her team used a computer model to simulate water molecules under pressure — with and without TMAO. That model used some of Yancey’s data showing how TMAO levels increase with depth.
Harrison Laurent is a physicist on the Leeds team. His group did more than just run a simulation, he says. The team checked that what the simulation modeled is as close as possible to what “actually happened” to the water at deep pressure.
To do this, the group used a second technique called neutron scattering. They blasted water samples with neutrons. That’s a type of subatomic particle. By measuring how neutrons bounce off the water molecules, they could learn how the water molecules were organized. Neutron scattering bridges the gap between computer simulation and reality, Laurent explains: “You’re getting the atomic resolution.” He says it shows how well reality compared to those computer-modeled data.
When TMAO was in the water, it bonded to the water molecules, the British group showed. That bonding stabilized the water’s structure. This kept the water from crushing — and deforming — the proteins. That could explain why the water no longer warps a fish’s proteins out of shape. Even under pressure, that water behaves almost as if it’s not under pressure.
Applications above sea level
This study helps “us to understand the natural limits of life,” says Dougan. But working out how molecules like TMAO work might be useful in other fields, too.
TMAO already has been tested in medicine, Yancey says. However, some of those trials are a bit creepy. In one 2009 study, for instance, Chinese researchers injected TMAO into the eyeballs of people with glaucoma. Glaucoma is a disease that increases pressure in the eye. The injections helped. TMAO decreased deformation of proteins in the eyeball. The proteins kept working normally. And that protected eyeball cells that otherwise might have died.
Other examples exist too. A 2003 study suggested that TMAO might treat cystic fibrosis. This lung disease is another “pressure problem,” Yancey says. It’s “a different kind of pressure” than undersea, but TMAO still helped. It supported the structure of a protein that usually doesn’t work in cystic fibrosis.
Yet TMAO treatments haven’t taken off. And Yancey suspects he knows why. You’d have to take so much TMAO into your body that you’d probably wind up smelling like rotten fish. However, he adds, TMAO is now being used for stabilizing some proteins in lab settings.
“The authors have really done a great job zooming in at what’s going on at the molecular level,” says Gerringer at SUNY. And they’ve shown how fish thrive in deep, ultra-high-pressure realms. That’s the home of the hadal snailfish. It’s one of the deepest living fish species on Earth.
“We often think of deep-sea fishes as being really toothy,” she says. But those creatures with big chompers are practically puddle-swimmers compared to the far-deeper-dwelling hadal snailfish. These deeper denizens are “adorable… almost fragile-looking,” she says. And “they are surprisingly and beautifully adapted to these [ocean] trench environments.” Now we understand better how they do that.