Here’s why scientists want a good quantum computer
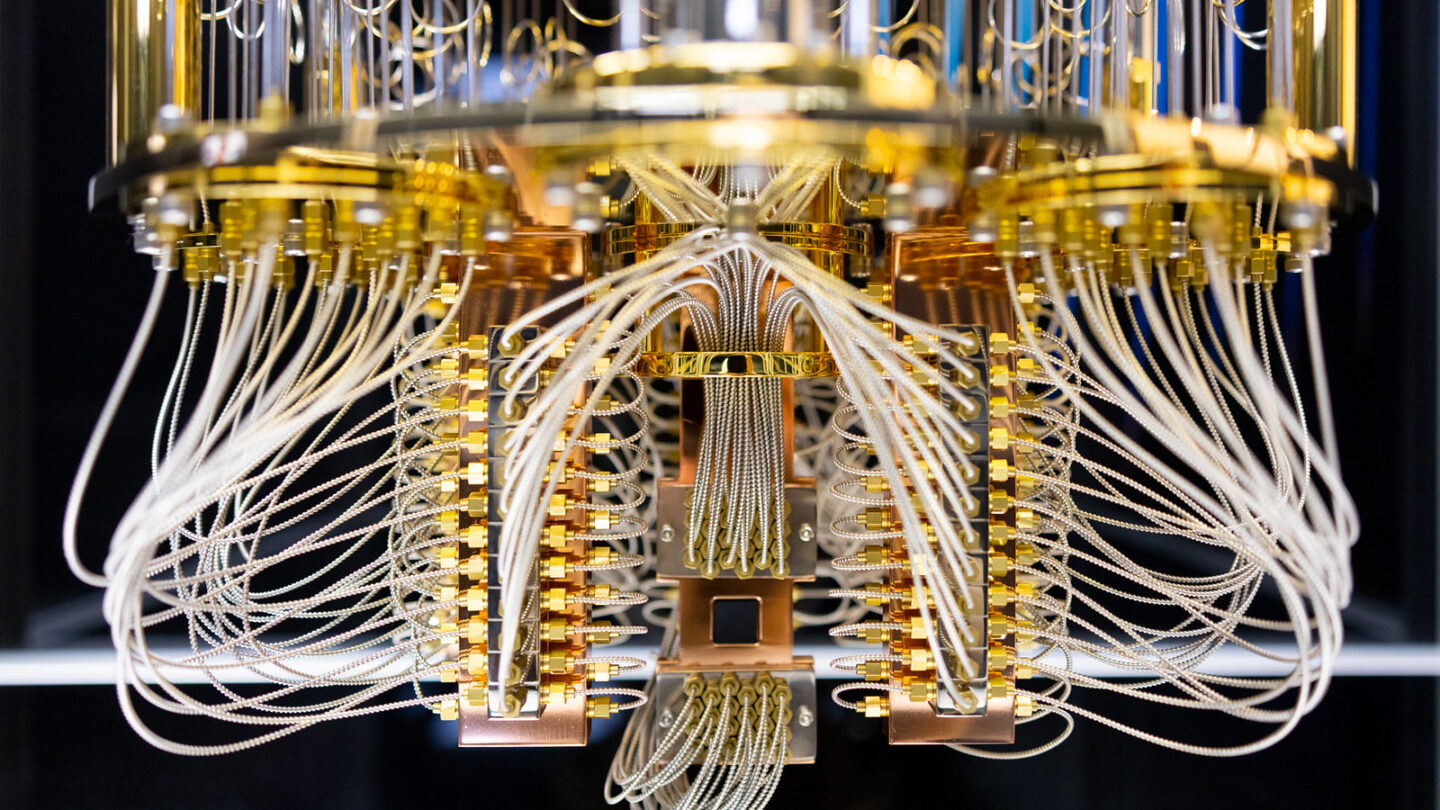
A quantum computer may look like a futuristic sculpture, but most of what you see is machinery used to keep the device a hair above absolute zero. The part that does the computing is the small rectangle near the bottom.
Satoshi Kawase, for IBM/IBM Research/Flickr (CC BY-ND 2.0 DEED)
In October 2022, U.S. President Joe Biden visited a quantum computer in Poughkeepsie, N.Y. The gorgeous contraption had been built by the high-tech company IBM. Like a futuristic sculpture, it had complex curves of gold tubes and wires.
Photos of the visit show Biden studying the machinery. But anyone looking at those photos might wonder: How is this thing a computer?
The temperature inside the machine, called IBM Quantum System One, is almost unfathomably cold. It’s a fraction of a degree above absolute zero, which is the coldest possible temperature. IBM has installed Quantum System One machines in many places around the world. Some of them are encased in the same kind of glass used to protect Leonardo da Vinci’s Mona Lisa.
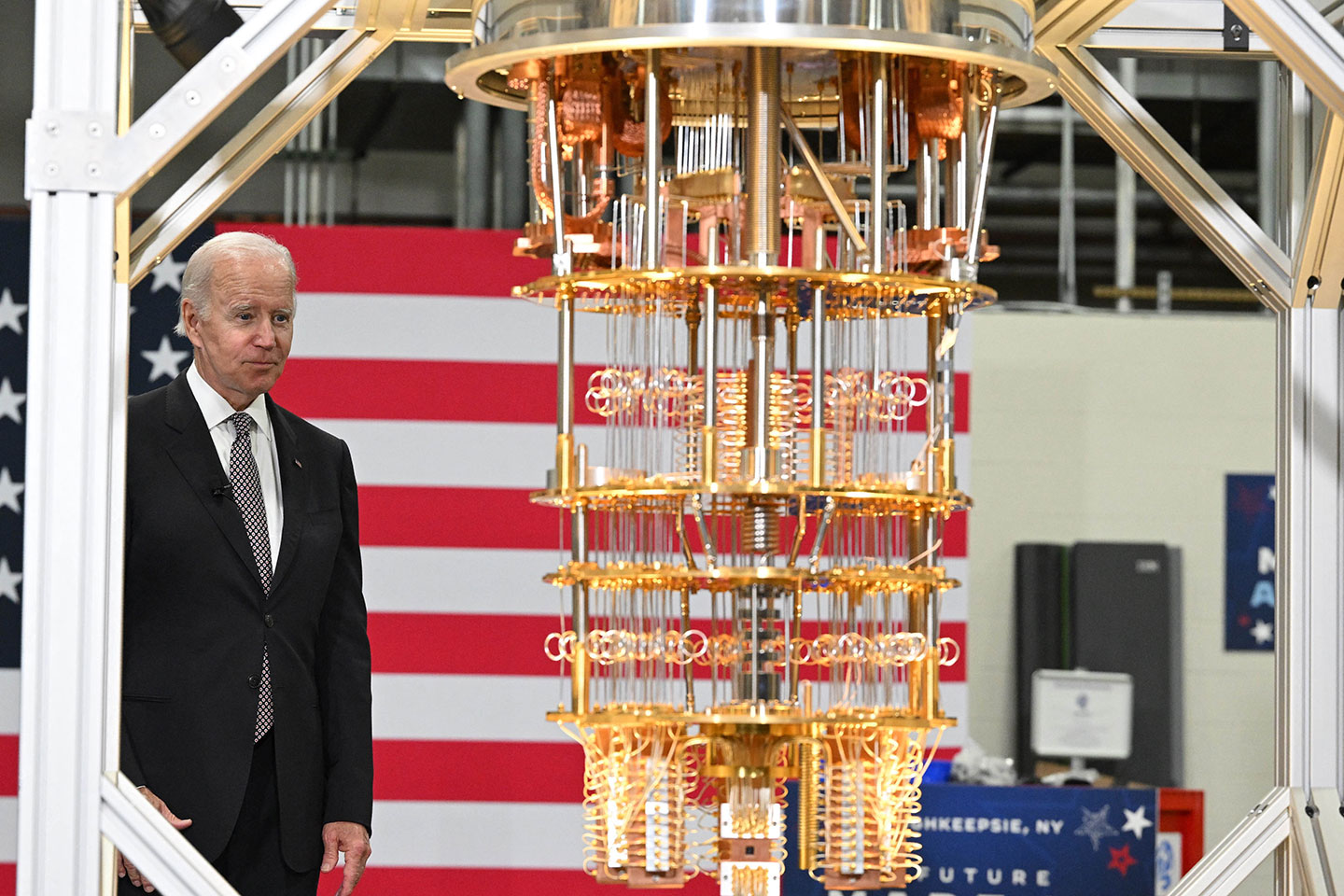
At absolute zero, everything stops moving. And quantum computing can take place only at a sliver of a fraction of a degree above absolute zero. The golden sculpture-looking part of Quantum System One is what keeps the computing part so cold.
“Any time you google ‘quantum computer,’ the pictures that come up are these big beautiful golden chandeliers,” said Corban Tillemann-Dick. He founded Maybell Quantum Industries in Denver, Colo. He spoke there about these quantum machines last February. It was at the annual meeting of the American Association for the Advancement of Science.
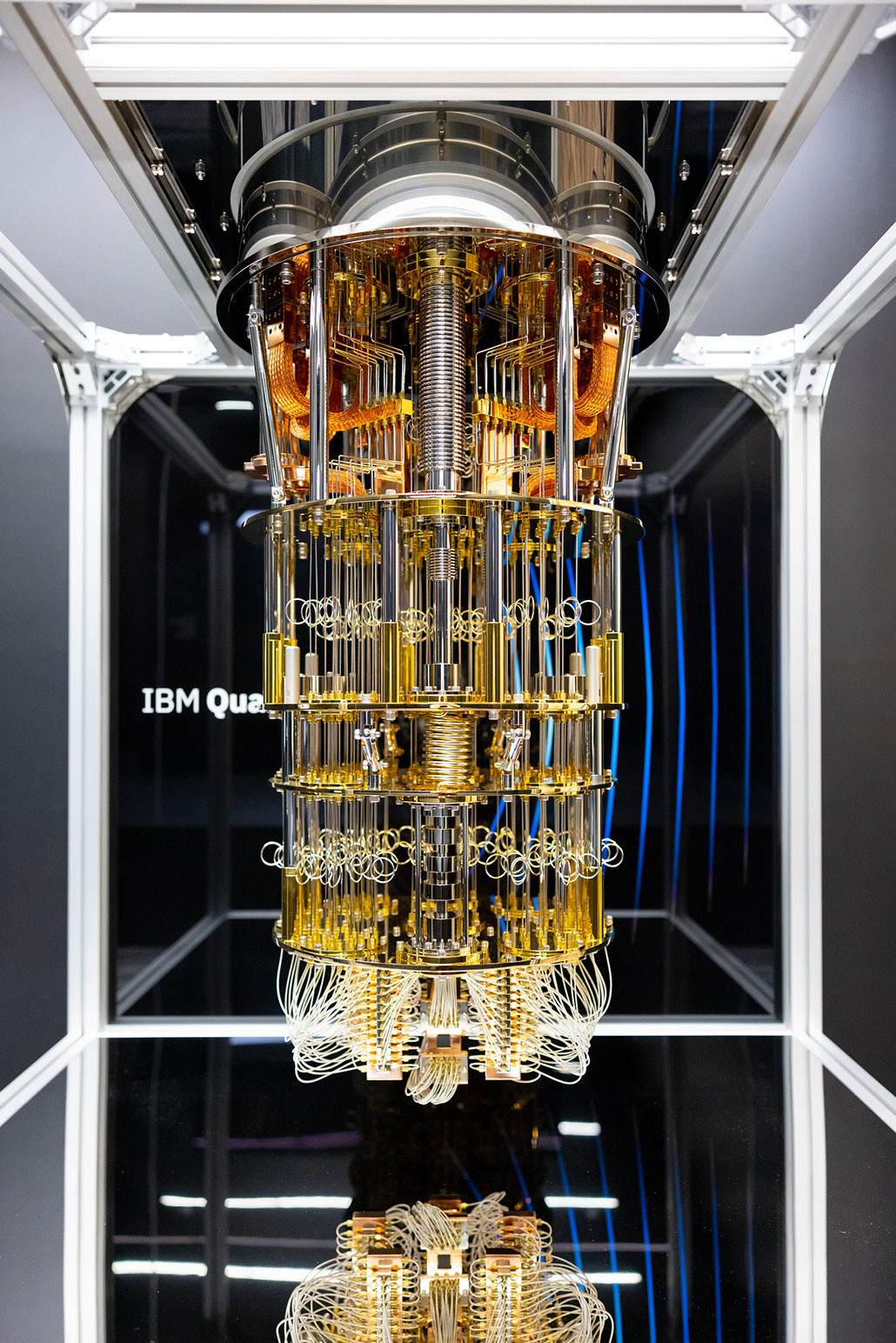
Looking at those photos, “I get kind of amused,” he said. “They think they’re standing next to a quantum computer. But really that chandelier is the fridge.”
The part that solves computing problems sits on a piece of silicon at the bottom of the contraption. It’s so small it could fit in your hand. Tillemann-Dick should know. He designs and builds ways to keep those quantum devices cold. (He jokes that he’s a high-tech refrigerator salesman.)
Tech companies — including IBM, Microsoft, Google and others — are racing to build the best quantum computer. Why? Their scientists think these devices will soon be able to complete tasks and solve problems faster than any number-cruncher today. Quantum computing will likely be able to tackle problems that today’s machines can’t.
Quantum computers are not able to do that yet. But they’re getting close.
Kayla Lee is a biologist by training who now works on building a global quantum-computing community at IBM in Charlotte, N.C. It’s an exciting time to be in the field, she says. “There’s so much opportunity.”
What’s the big deal?
We’ve already gotten some glimpses that quantum computers might one day outperform normal, or classical, computers.
Take Sycamore. Last December, Google reported that Sycamore — its quantum system — had quickly completed a difficult task related to how a random system runs. It required only six seconds. The same task would likely require years on the world’s fastest traditional computer. That’s a supercomputer known as Frontier. It’s housed at the Oak Ridge National Laboratory in Tennessee.
Google’s test put Sycamore through some early paces. The problem it solved in six seconds showed off the machine’s number-crunching power. But that computing task itself isn’t very useful for solving the world’s problems. No quantum computer works well enough yet to start solving big, important challenges. What’s got experts excited is what this new generation of computers might do — and soon.
Some predict quantum machines will solve complex problems related to climate change. Quantum computers might also identify better battery designs. They might find new ways to generate renewable energy or make energy systems more efficient.
Quantum computers could also help solve what seem like old-school problems, but really aren’t.
Take figuring out how to move goods around the world. This is remarkably hard to optimize because it involves trucks, trains, ships and planes moving across different environments. Shipping companies can save time, money and energy by using the best routes. And quantum computers may help find those paths.
Part of Lee’s job at IBM is to help people learn what quantum computers will and won’t be able to do. She also helps businesses and researchers get started in learning how to use such a device. Even though it’s a computer, a quantum computer is unlike the technology behind laptops, phones and other electronics.
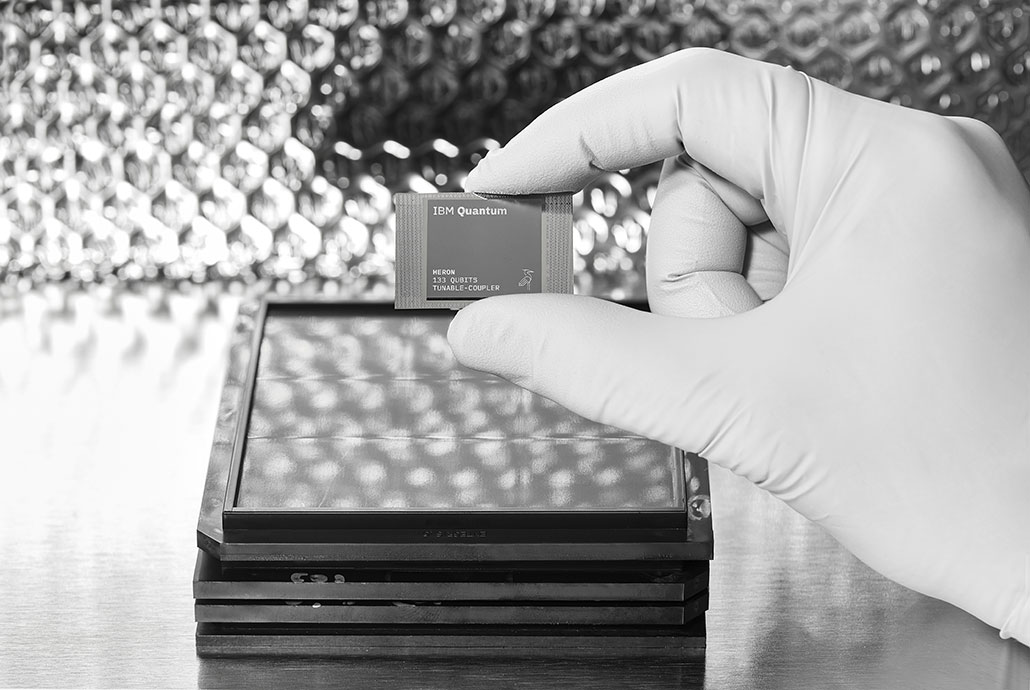
Some news articles, Lee says, oversell the possibilities. “It’s not just a faster computer,” she says. “It’s not going to solve every problem.”
One big challenge is to find the problems that quantum computers will solve faster.
In some cases, a quantum computer may not offer an advantage if there’s no quantum algorithm, or recipe, for solving a problem. And some problems solved by quantum algorithms can still be solved as fast by classical computers. Quantum computers also may not be good at tasks that require lots of memory, because they can’t yet store much data. They also may not speed up tasks that focus on multiplying or adding numbers because classical computers already do that well.
But these emerging computers should excel at certain types of problems, Lee says. Knowing how depends on knowing how quantum computers work.
Quantum weirdness
The science that led to quantum computing goes back hundreds of years. It started with physicists who wanted to know more about the behavior of light.
In the 17th century, Isaac Newton argued that light was made up of tiny particles. But experiments in the 18th and 19th centuries showed that light traveled like a wave. By 1900, most scientists thought light was made up of waves.
In fact, both Team Wave and Team Particle turned out to be right — and wrong.
In 1905, physicist Albert Einstein put the pieces together. He proposed that light traveled like a wave, but it could be observed like a particle. Another physicist later introduced the term “photon” for this type of particle.
Surprising discoveries about photons in the early 1900s paved the way for quantum computers, Lee says. Key among these was the idea of superposition.
The principle of superposition says that thanks to its wavelike nature, a photon can exist in more than one state at one time. Here, the word “state” describes some physical property of the particle, such as its location. So the rule of superposition says that a photon can be in more than one place at a time.
We will never actually see a photon in two states (such as two places) at once. As soon as you look at a photon, it only has one location. Physicists explain this by saying it “collapses” into one state. But while a photon remains unobserved, superposition allows it to exist in multiple states at once.
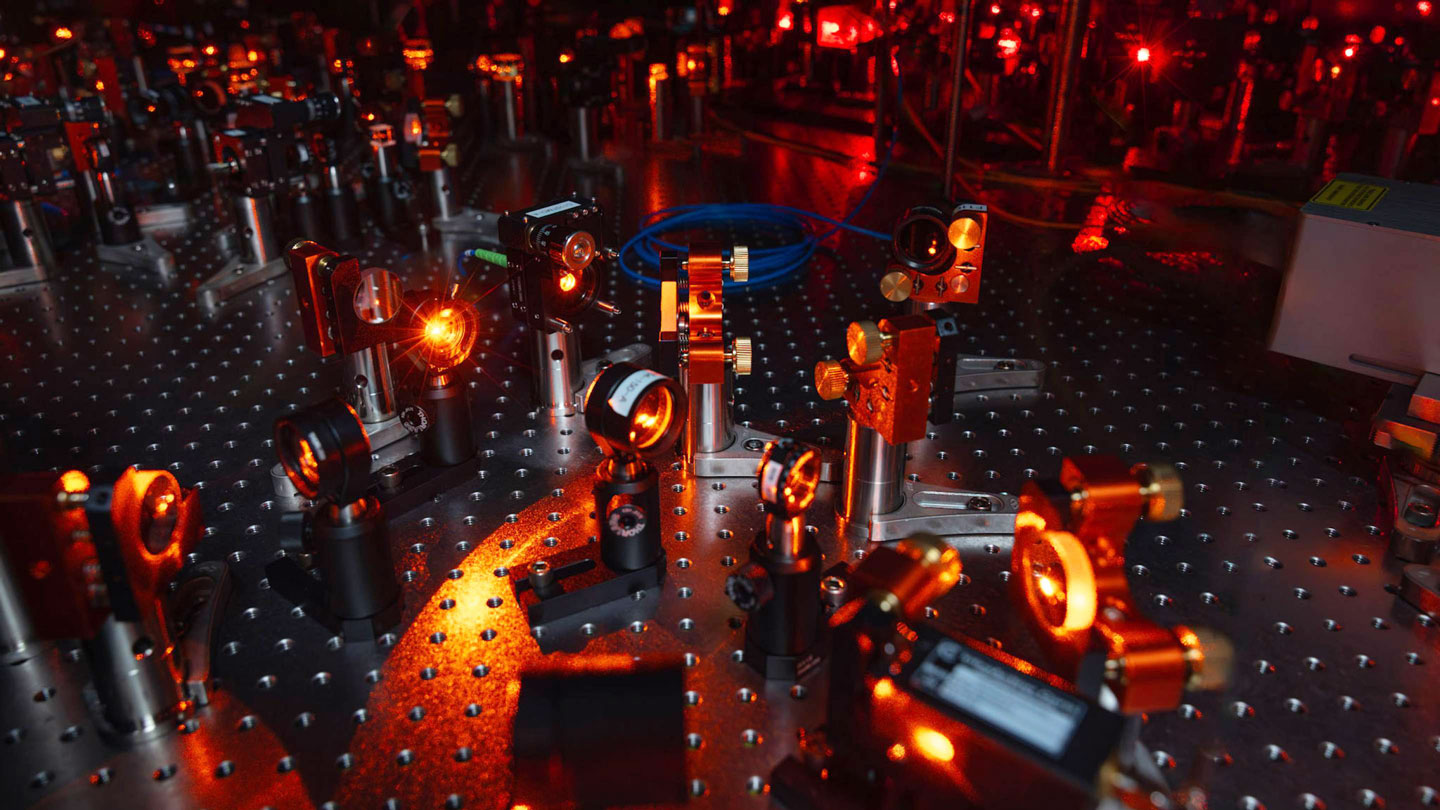
Physicists have since discovered that photons are not the only particles that can act like waves. Electrons, atoms and even molecules can show wavelike behavior. So they, too, follow the rule of superposition. It’s just harder to see these weird quantum effects as you look at bigger objects at warmer temperatures.
If that sounds confusing to you, you’re in good company.
“I think I can safely say no one understands quantum mechanics,” Richard Feynman said in a lecture he gave in 1964. And he should know. He’s the physicist who came up with the idea to harness the weirdness of superposition in a computer.
Even after scientists and engineers like Feynman had the idea for a quantum computer, it took them decades to build such a machine. Think of it, Lee says, as “a new model of computation.”
Bits, qubits and entanglement
A normal computer stores and processes information using bits. A bit is a sort of switch that can be in one of two states: on or off. This can be described as the bit having a value of “1” or “0.” Each bit must be one state or the other at any given time.
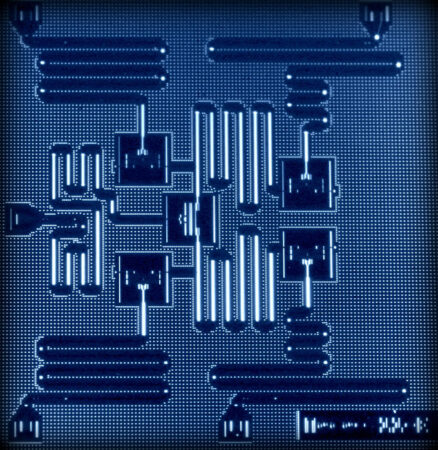
If you’re reading this on a screen, then every letter, number and punctuation mark you see is represented inside your digital device by bits. It requires about 5 bits to represent a letter or number. Inside a computer, a device called a transistor acts as the bit. Modern computers can hold hundreds of billions of bits.
Quantum computers are different. Instead of bits, quantum machines store and process data using qubits. That’s short for “quantum bit.” Qubits can be made from any quantum particle or molecule that can exist in a superposition of states.
Unlike a bit, a qubit isn’t limited to just being on or off. Because of superposition, it has some probability of being on and some probability of being off at any given time. It doesn’t settle into one of those states until someone measures it. Then, it “collapses” into a single state, just like a photon does when someone observes it. The combination of all those collapsed qubits reveals the answer to the problem.
“That’s what gives us the big speed up,” says Dan Gauthier. He’s a physicist at the Ohio State University in Columbus. His research focuses on how to use quantum technology in new tools.
Because they might be in multiple states at once, qubits can process data faster than classical bits, Gauthier says. Imagine you wanted to find your way through a maze. Using a classical computer is like trying each possible path one at a time. A quantum computer, on the other hand, acts more like a mist that can float through walls, hover over all the ways through the maze and condense into the correct path.
A bunch of connected qubits can pack a lot of computing power. Say you have a quantum computer and an ordinary computer that can store and compute the same amount of information. If you add just one qubit to the quantum computer, your ordinary computer will now need twice as much memory to store the same information as the quantum one. It will require twice as much time to compute the same problem, too.
Quantum computers may also get a boost from another strange feature of physics. It’s called entanglement. Physicists predicted this special kind of connection between photons in the 1930s. If two photons are entangled, each loses its individuality. The two now share a single set of properties. If scientists measure the properties of one particle, they automatically know those same properties of the other particle.
The oddest part of entanglement? If you entangle two particles and take them far away from each other, they remain entangled. This has been proven in lab experiments. Albert Einstein famously called this “spooky action at a distance.” Why? Because it seems like the particles somehow share information instantly. And nothing, not even information, is supposed to travel faster than the speed of light.
How entanglement works is still a physics mystery. But scientists have found ways to entangle photons. They’ve found ways to entangle atoms, too. In December 2023, physicists at Princeton University even showed how to entangle molecules made up of many atoms. Entanglement could be useful for quantum computers because errors are easier to find and correct in qubits with entangled states.
Scientists are still trying to find the best ways to build qubits and to put them together. The challenge, explains Gauthier, is working with such small things. Superposition and entanglement can only be observed at tiny scales and very cold temperatures. “Quantum mechanics has different rules,” he says, “about how we make a larger system from a bunch of small quantum entities.”
Solving problems the quantum way
Here’s one example of a problem that a quantum computer might solve.
Imagine your math teacher gives you two very large prime numbers and asks you to multiply them together. (Remember that a prime number is divisible only by itself and 1.) You can probably do that pretty easily, especially with a calculator.
Now imagine you’re given the same problem in reverse. Your teacher gives you a very large number and asks you to find two prime numbers (factors) that when multiplied together will give you that big number.
It’s more difficult. You could start by trying to divide the large number by prime numbers, moving up the list number by number. Divide by 2, then 3, then 5, then 7 and so on. But a calculator won’t help you much. Neither will a regular computer. Even with a good algorithm this task becomes very hard, very fast. Finding factors for a 250-digit number would require thousands of years on an ordinary computer.
Do you have a science question? We can help!
Submit your question here, and we might answer it an upcoming issue of Science News Explores
Not so for a quantum computer!
Because of quantum superposition, this device could more quickly rule out wrong solutions and find the right ones. In 1994, mathematician Peter Shor described a quantum algorithm for how to do it. Mathematicians think Shor’s algorithm offers a speedup because of entanglement. A powerful enough quantum computer could identify those factors quickly. It can evaluate all solutions at once, explains Gauthier.
The ability to find prime factors of super large numbers may not sound useful. But it could actually pose a privacy risk to lots of sensitive information sent over the internet. That may include financial or health records.
When data is sent online, it is encrypted — or hidden from hackers — using an algorithm. One of the most powerful algorithms for encryption involves multiplying large prime numbers. In order to make sense of encrypted data, the intended recipient has to know those two numbers. They act as a sort of key for unlocking the message.
Onlookers may be able to see the encrypted message. But to make sense of it, they’d need to know those two prime factors. And since finding those factors is a hard problem for any computer today, no hackers should be able to find the key.
With Shor’s algorithm, a quantum computer could. Then, a hacker could unmask the encrypted data. This makes computer security experts nervous. So computer scientists are on the hunt for quantum-safe ways to keep our data protected.
Lee, at IBM, points out that quantum computers will be able to solve other real-world, complex problems too. They might be used to find new medicines. Or study financial systems like the stock market. Or analyze chemical reactions — or even help scientists better understand quantum physics.
Plus, remember the problem of finding the most efficient routes for transporting goods? Using qubits, a quantum computer could quickly find routes that were cheaper, faster or more energy-efficient or that had a smaller carbon footprint. Or it could find a better mashup of all of these.
What’s stopping the quantum computing revolution?
Right now, all of these applications remain just possibilities. They can’t be calculated yet, at least not in any reasonable amount of time. Quantum computers also don’t yet have enough qubits — or enough accuracy — to factor enormous prime numbers. So they don’t yet pose a risk to encrypted data.
In fact, today’s qubits are prone to errors. That’s because they’re susceptible to noise. Noise can be anything that interferes with their superposition. Wi-Fi signals, for instance, or temperature.
Noise mixes up the information that’s stored or computed in a quantum computer. A large part of quantum computer research focuses on error reduction. This can be done by changing the design of quantum computing chips, improving software or better harnessing entanglement.
“We’re still figuring out what a good qubit looks like and how do they talk to each other,” says Lee.
Companies are racing to build bigger, better quantum computers. In October 2023, a U.S. company called Atom Computing unveiled the first one to have more than 1,000 qubits. IBM has also since built a 1,000-qubit machine. The latest version of Google’s Sycamore system has only 70 qubits, but they produce few errors.
Researchers are excited by the fast progress and the potential of these systems. But quantum computers need some time and tests before they’ll be truly useful.
“If you ask me when we will have any real payback, I have no idea,” Gauthier says. “I’m always amazed at how quickly things are progressing. But I’m still unwilling to put a real time scale on it.”