Explainer: How our eyes make sense of light
Special cells send data describing a scene into the brain, which interprets it
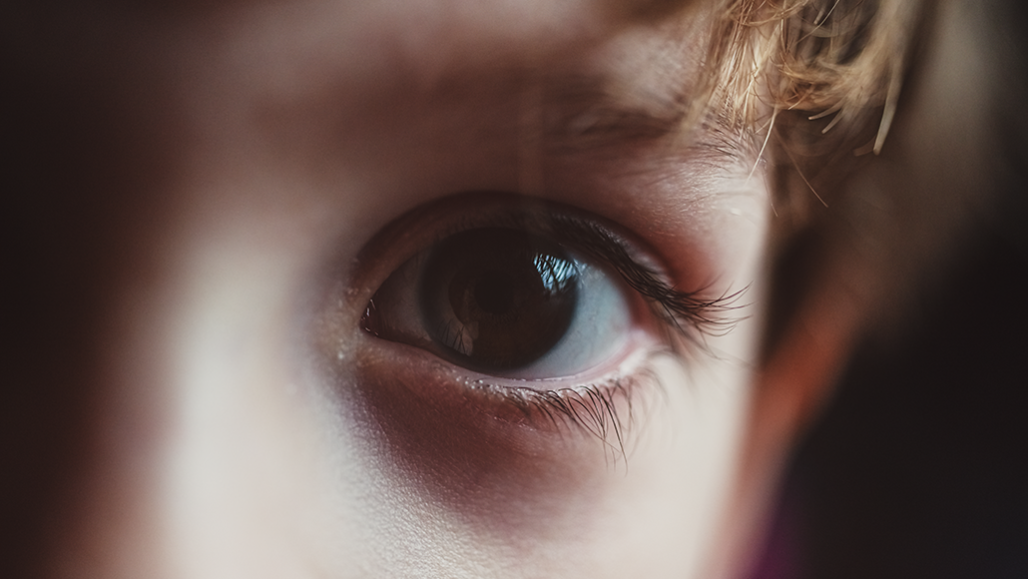
What we see of the eyes from the outside offers few clues to the light-interpreting operations at work inside.
Shaunl/E+/Getty Images
How do your eyes work? It’s far more than just forming a tiny picture in your eye. There’s also color and motion. It takes many cells — and finally the brain — to make sense of it all.
As light enters our eyes, it first heads through a tough outer tissue called the cornea. This protects the delicate inner eye from everything the world might throw at it. Light passes right through the cornea and into a transparent, flexible tissue called the lens. This lens focuses the light, sending it through the liquid-filled globe of the eyeball to the back interior wall of the eye.
The tissue there, known as the retina, contains millions of light-sensitive cells. They are especially concentrated in an area called the fovea (FOH-vee-ah). This densely packed set of cells gives us the clearest picture of our world. When the eye focuses on an object, it directs the light bouncing off the object directly onto the fovea to get the best image. In fact, when the eye focuses on something, that’s called foveating (FOH-vee-ayt-ing).
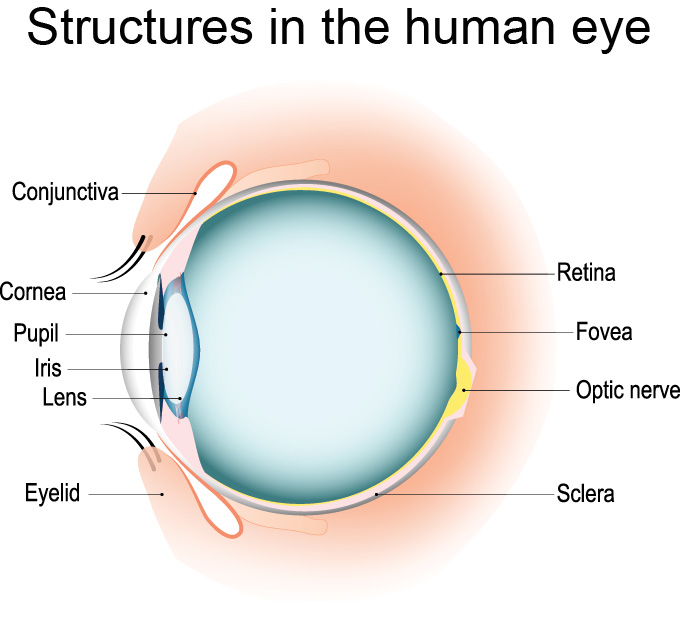
The light-sensing cells on the retina are known as photoreceptors. Two important types are rods and cones. Each human retina (and you have two, one in each eye) contains 125 million rods and about 6 million cones. This is 70 percent of all the sensory receptors in your entire body — for touch, taste smell, hearing and sight all put together. That’s how important vision is to us.
I’ll take a cone, please
Each rod or cone cell at the back of the eye has a stack of discs inside, The discs contain a pigment molecule. It’s bound to a protein called an opsin. Rods and cones each have a different opsin.
Cones have a pigment-protein pair called photopsin (Foh-TOP-sin). It comes in three different types, and each cone has just one type. They come in red, green or blue — the colors that each cone type is best at absorbing. Cones respond to light that has passed through the lens and onto the fovea. As each cone absorbs its color of light, it produces an electrical signal. These signals travel to the brain, filling our worlds with color.
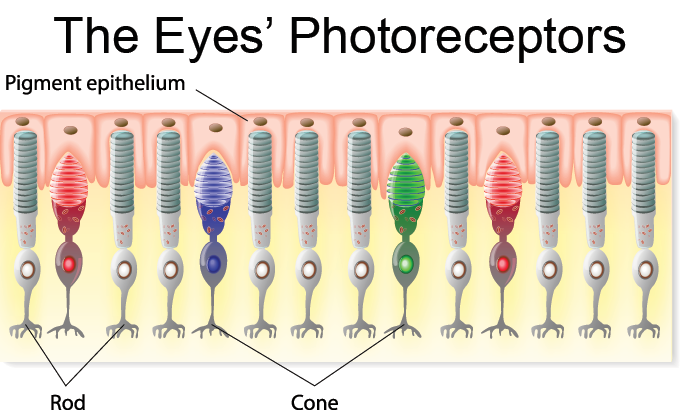
In September 2016, a vision researcher at the University of Washington in Seattle discovered some cones also sense white light. But only white light. That was a big surprise, Ramkumar Sabesan said at the time.
In fact, he and his colleagues found, so-called red and green cone cells each come in two types. One transmits white light, the other relays color. Especially surprising, most of these cones are the white type. Out of 167 red cones tested, 119 signaled white. Of 98 green cones tested, 77 reported white light. (The team didn’t test white sensitivity among the retina’s few blue cones.)
White-sensing cells also detect black (which is the absence of white). The data they relay create a sharp black-and-white picture of someone’s surroundings. These provide a crisp edge to visual details. Red- and green-signaling cells fill in the lines with blurrier chunks of color. The process, says Sabesan, works much like filling in a coloring book or adding color to a black-and-white film.
Red, green, blue, black and white. These five colors end up making every single color that we see. Cone cells are especially concentrated in the fovea, and work only in bright light. At night, you need your rods.
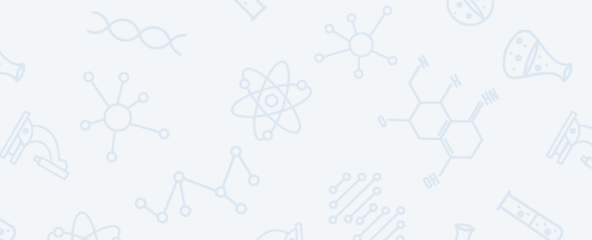
Educators and Parents, Sign Up for The Cheat Sheet
Weekly updates to help you use Science News Explores in the learning environment
Thank you for signing up!
There was a problem signing you up.
Rods are on the dark side
The retina’s rod cells aren’t part of the cone coloring system. They work when light levels are low. Instead of photopsins, rods have a different pigment-protein pair: rhodopsin (Roh-DOP-sin). Rods produce images only in shades of grey. But they are much more sensitive to light than cones are. They are so sensitive that a rod cell can detect a single photon of light — the smallest possible particle.
In the dark, we rely on our rods. But light inactivates these cells. It stimulates them so much that they become unresponsive. That’s all right, cones are there to take over. They require much more light to function. So we rely on cones in the light.
When they detect certain wavelengths of visible light, the photoreceptors trigger electrical signals. Rods and cones will send these signals through nerves that reach into the brain. They head for the occipital (Awe-SIP-ih-tal) cortex, right up against the back of the skull. There, the brain interprets these signals to make sense of what we’re looking at.
The retina also is home to another type of light-sensitive cell. These melanopsin ganglion (MEH-lah-NOP-sin GANG-lee-un) cells don’t send signals to the occipital cortex. Instead, they report the presence of light to the olivary pretectal nucleus (OH-liv-airy Pree-TEK-tahl NEW-klee-us). This is a tiny spot in the middle of the base of the brain. The signals that melanopsin ganglion cells send here help regulate the body’s master biological clock. They also send signals that control the size of the pupil (which controls how much light gets into the eye in the first place).
Light signals sent to this master body clock tell you when to be sleepy and when to be alert. But not just any light will do. This clock can distinguish between different colors of light. Blue works best to stimulate the body clock. Sunlight is an excellent source of blue light. Although it looks white, sunlight actually is a mix of many colors, including blue. This might explain why stepping outside on a bright sunny day helps clear the fog from your head.