Explainer: Reflection, refraction and the power of lenses
From eyeglasses to microscopes, two laws of physics work light-bending magic
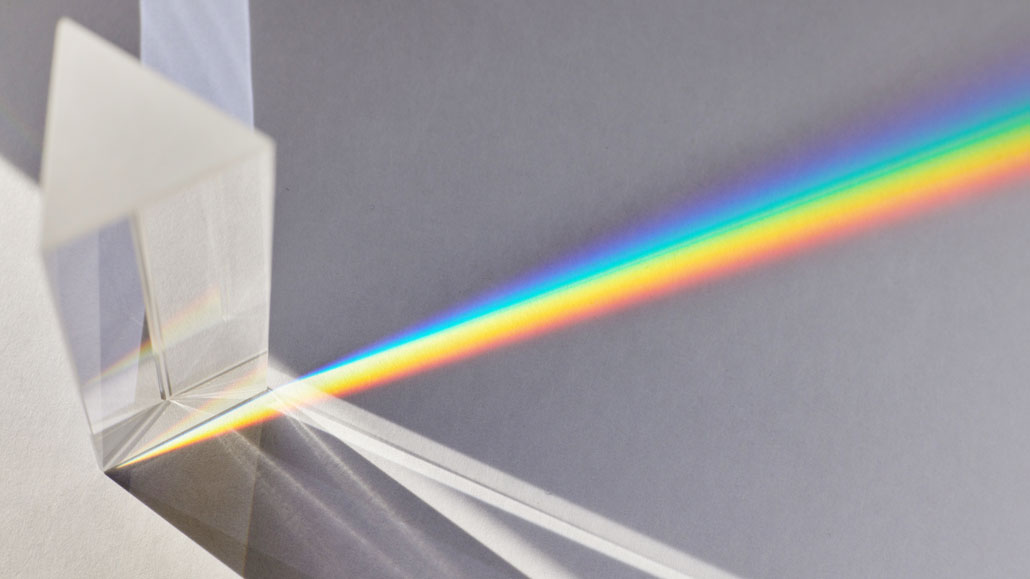
A prism causes incoming light to bend by different amounts, depending on the wavelength — or color — of that light. As light passes through the prism and exits on the other side, it spreads out into a rainbow.
Ekaterina Demidova/Moment/Getty Images
By Trisha Muro
Microscopes, telescopes and eyeglasses. All of these work by manipulating the movement of light.
When waves of light hit a smooth surface, such as a mirror, they reflect off of it. They also bend, or refract, when they move between environments of different densities, such as when light passes from air into and through a glass lens. Together, these basic properties of light allow scientists to design lenses and mirrors to suit their needs — whether it’s to peer across the cosmos or deep inside a cell.
Reflection
Look in a mirror and you’ll see your reflection. The law of reflection is simple: Whatever angle a beam of light makes as it collides with a mirror is the same angle it will have as it bounces off the mirror’s surface. If you shine a flashlight at a 45-degree angle onto your bathroom mirror, it will bounce off at a 45-degree angle. When you see your reflection, the light shining on your illuminated face hits the mirror dead-on, so it bounces right back to your eyes.
This only works because a mirror is a polished surface that’s extremely smooth — and therefore reflective. Its smoothness makes all of the light that hits it from a certain angle bounce off in the same direction. The surface of a painted wall in your bedroom, in contrast, is so bumpy that it doesn’t reflect very well. Light that hits the wall will reflect off those bumps, bouncing off in a mix of different directions. That’s why most walls look dull, not shiny.
You might have noticed that inside flashlights and headlights, there’s a single, small light bulb with a curved mirror behind it. That curve collects the light coming off the bulb in many different directions and focuses it into a strong beam that leaves in one direction: outward. Curved mirrors are extremely effective at focusing beams of light.
A telescope’s mirror works the same way. It focuses the incoming light waves from a distant object, like a star, into a single point of light that’s now bright enough for an astronomer to see.
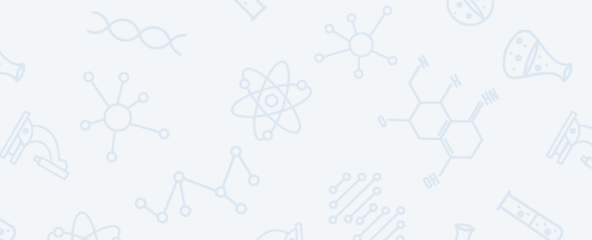
Educators and Parents, Sign Up for The Cheat Sheet
Weekly updates to help you use Science News Explores in the learning environment
Thank you for signing up!
There was a problem signing you up.
Refraction and rainbows
You know how a straw appears to bend as it sits in a glass of water? That’s due to refraction. The law of refraction states that light waves will bend when they move from one medium (such as air) to another (such as water or glass). This is because each medium has a different density, also known as its “optical thickness.”
Imagine running along a beach. If you start running on a concrete path, you can sprint fairly quickly. As soon as you cross onto sand, you slow down. Even if you’re trying to move your feet at the same speed as before, you can’t. You’ll slow even more as you try to keep running through the water. The “thickness” of each surface you’re now running through — sand or water — slows you down compared to when your feet were moving through air.
Light, too, changes speed in different mediums. And since light travels in waves, those waves will bend as they change their speed.
Back to that straw in a glass of water: If you look through the side of the glass, the straw will look like a zigzag. Or, if you’ve ever placed a diving ring at the bottom of a shallow pool and attempted to grab it, you’ll have noticed the ring isn’t exactly where it appears to be. The bending of light rays causes the ring to look as if it’s located a short distance from its actual spot.
The effects of this bending are greater or smaller depending on the light’s wavelength, or color. Shorter wavelengths, such as blue and violet, bend more than longer ones, such as red.
This is what causes the rainbow effect as light passes through a prism. It also explains why red is always the uppermost color in a rainbow and violet the lowermost hue. White light entering the prism contains all different colors of light. Red light waves bend the least, so their path stays closer to a straight line. That leaves red at the top of the rainbow. Violet light waves bend the most when passing through the prism, so that hue dips down to the bottom. The other colors of the rainbow end up in between red and violet, based on how much their waves bend.
Reflection + refraction
Reflection and refraction can work together — often with awesome results. Consider the bending of the sun’s light as it passes through Earth’s atmosphere at a low angle. This tends to happen at sunrise or sunset. Sunlight’s bending, or refracting, paints clouds near the horizon in an array of red and orange hues.
You may have also noticed that the most spectacular sunsets happen when the air is either dusty or moist. In those cases, sunlight is refracted by Earth’s atmosphere and reflected around by particles of dust and water vapor.
The same thing happens in rainbows. As sunlight enters each individual raindrop, the ray of light refracts as it moves from the air to the water of the droplet. Once inside the raindrop, the light actually reflects off the inside of the drop. It bounces once, then begins to head back out of the raindrop. But as the light passes from inside the drop back into the air again, it refracts one more time.
That’s two refractions plus one internal reflection.
Light passing through raindrops forms a rainbow’s distinct arc for the same reason light passing through a prism does. Red forms the outermost arc and blue the innermost one. As the colors splay out, we get to delight in the beauty of those smeared hues. (A double rainbow happens when the light bounces twice inside each raindrop. Two refractions plus two internal reflections. That reverses the order of the colors in the second rainbow.)
Have you ever wondered why we don’t see rainbows in the snow like we do in rain? Maybe it makes sense now. Rainbows depend on the almost-spherical shape of water droplets. Snow is water, too, but its crystals have a completely different shape. That’s why snow can’t produce the same refraction-reflection-refraction pattern that raindrops do.
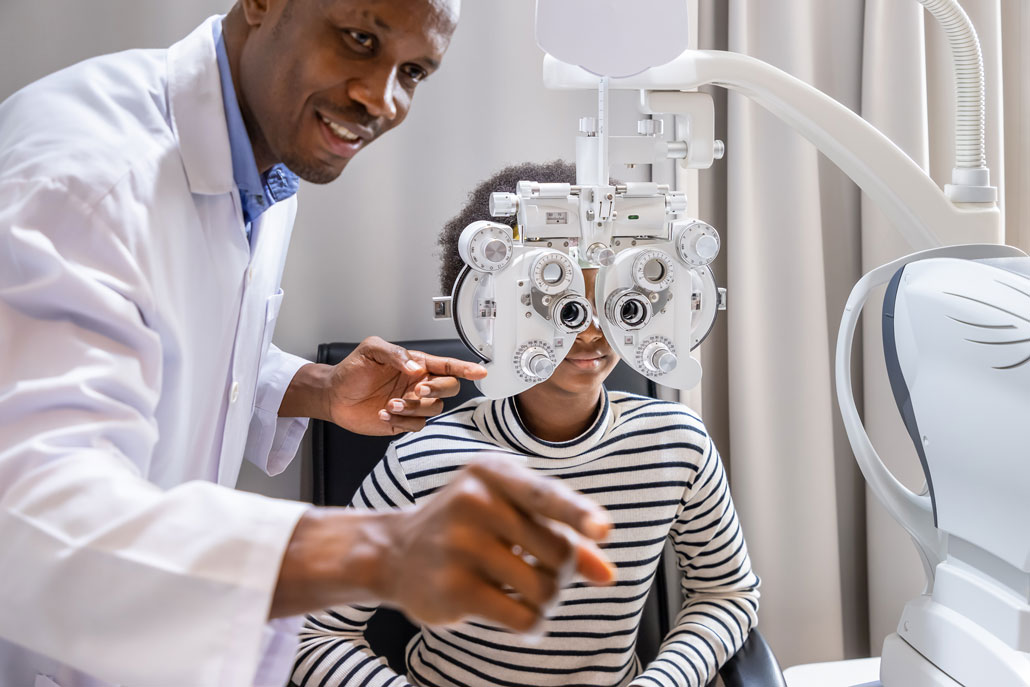
Lenses and mirrors
Lenses are tools that take advantage of light’s ability to bend. By carefully shaping a piece of glass, optical scientists can design lenses that focus light to make clear images. To magnify the appearance of an object, designers often combine a series of lenses.
Most lenses are made from glass that has been ground into a very precise shape with a smooth surface. The starting slab of glass looks like a thick pancake. By the time it’s ground into a lens, its shape will be very different.
Convex lenses are thicker in the middle than at their edges. They bend an incoming beam of light to a single focal point.
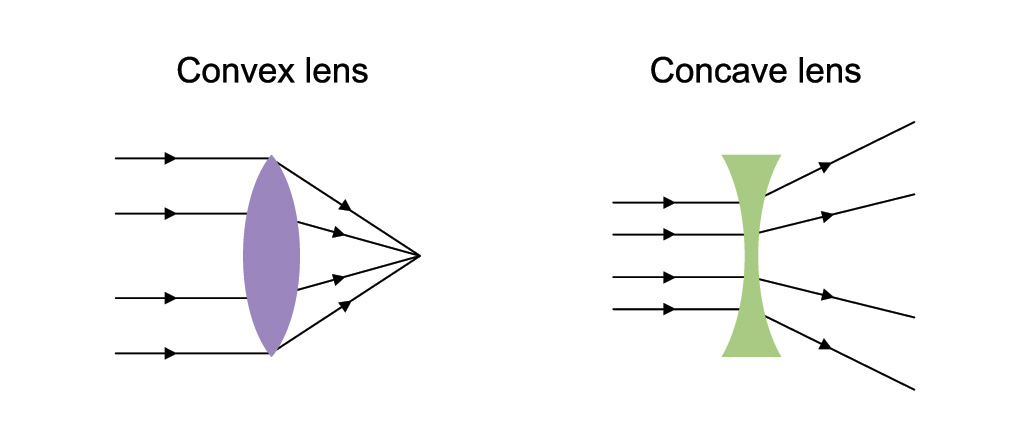
Concave lenses do the opposite. Thicker on the outside than at their center, they spread out a beam of light. Both types of lenses are useful in microscopes, telescopes, binoculars and eyeglasses. Combinations of these shapes allow optical scientists to direct a beam of light into any path that’s needed.
Mirrors, too, can be shaped to modify the path light takes. If you’ve ever looked at your reflection in carnival mirrors, they might have made you appear tall and skinny, short and rounded or distorted in other ways.
Combining mirrors and lenses can also create powerful shafts of light, such as those beamed by a lighthouse.
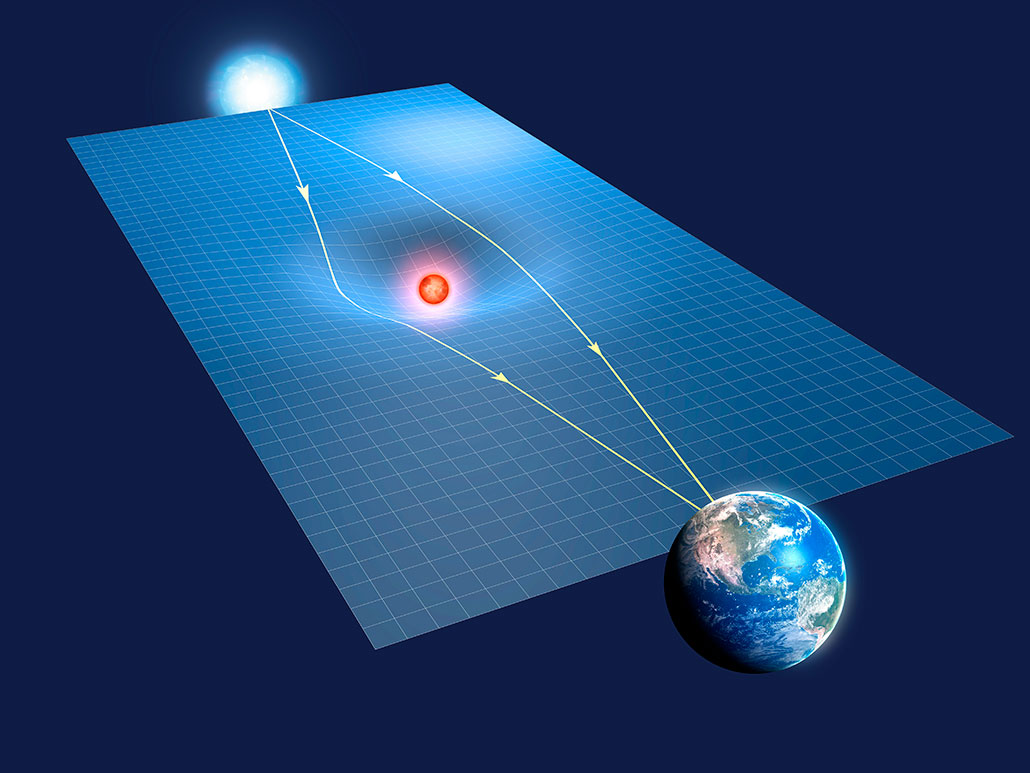
Gravity’s optical tricks
In one of the universe’s most magnificent tricks, intense gravity can act like a lens.
If an extremely massive object — such as a galaxy or a black hole — lies between an astronomer and the distant star they are looking at, that star can appear to be in a false spot (much like the ring at the bottom of a pool). The mass of the galaxy actually warps the space around it. As a result, the beam of light from that distant star bends with the space it’s moving through. The star might now even show up on the astronomer’s image as multiple identical appearances of itself. Or it might look like smeared arcs of light. Sometimes, if the alignment is just right, that light can form a perfect circle.
It’s just as weird as the light tricks of a funhouse mirror — but on a cosmic scale.