Photons map the atomic scale to help medicine and more
Researchers at a gigantic lab — the Advanced Photon Source — are probing the world of the very small
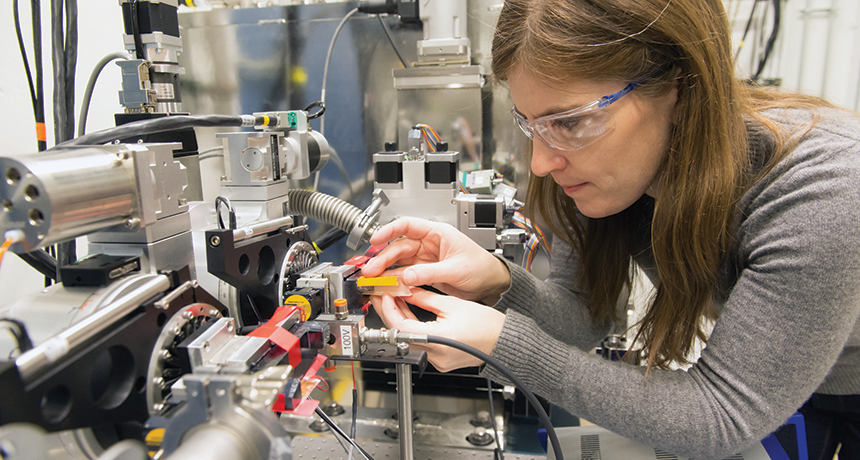
Physicist Mary Upton lines up equipment just right in preparation for an experiment using X-rays emitted by the Advanced Photon Source.
Argonne National Laboratory
A giant ring-shaped research laboratory in Illinois is providing a new window into teeny, tiny molecules. What scientists learn there can help them study diseases, build better batteries, design bridges and aircraft, fight pollution and more.
Known as the Advanced Photon Source, or APS, it sprawls across 8.6 hectares (more than 21 acres) at Argonne National Laboratory, just west of Chicago. This research center is big, both in terms of its value to science and its size.
The outer diameter of its experiment hall spans 373 meters — the length of three and a half U.S. football fields. Within the building is a ring of narrow pipes that measure 1,104 meters (3,622 feet) around. You could fit a Major League Baseball stadium inside this ring!
This facility is one of several particle accelerators around the world. Their goal is to send a beam of subatomic particles into some target and then watch what happens. (Subatomic particles are bits of matter smaller than atoms.) Those collisions can provide detailed information about the structure of things too small to see — things almost too small to imagine.
Some accelerators shoot their particles down long, straight lines. Others, such as this one at Argonne, send particles around and around some giant ring-shaped structure. And at APS, it’s not the beam of electrons that the scientists want to use — at least not directly. Rather, they want to harness the high-energy particles of light, called photons, which its electron beam creates.
Story continues below image.
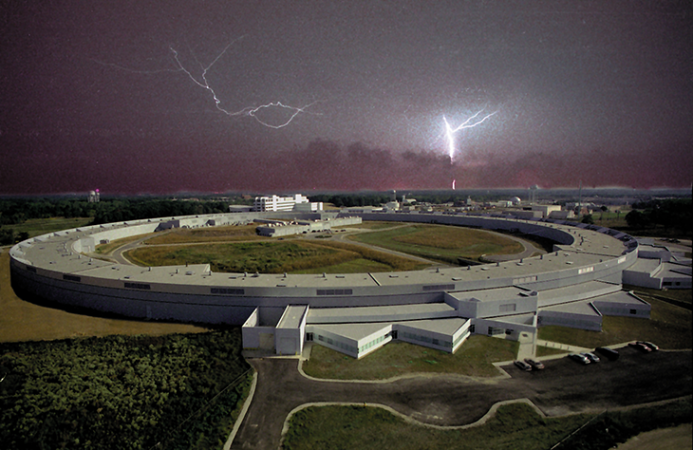
The photons’ high energy and tiny size are a powerful combo. Together they help scientists probe the properties of different molecules. In one recent study there, researchers showed how cooling a compound changes its ability to conduct electricity. In another, scientists revealed the point of attack for a disease-causing germ, called the Lassa virus. These teams and others can now use those findings for further work.
Clearly, argues APS physicist Mary Upton, this big, big facility is “a very special and unique tool” to understand the world of the very, very small.
Round and round they go
Left alone, a beam of electrons would travel in a straight line. Bending their path to go round and round takes some steering. At Advanced Photon Source, magnets do that job.
Equipment in a center building shoots out a beam of electrons. The accelerator then speeds up these electrons and steers them into a narrow pipe that runs through its large outer ring. Clusters of powerful magnets around the ring force these electrons to turn, a tiny bit at a time. Their path ends up making a big circle where they zoom around and around at nearly the speed of light. How nearly? Try more than 99.99999 percent of that ultimate speed limit.
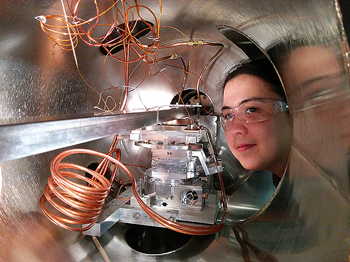
Other magnets around the ring steer the beam in more complex ways. These magnets are arranged with alternating pole positions. One steering magnet may have the north pole on top and the south pole on the bottom. The next would have the south pole on top and the north on the bottom. And so on. (The north and south poles tell which of the Earth’s magnetic poles the end of a magnet would seek if it were allowed to float freely. The north pole on a bar magnet would be attracted to Earth’s magnetic north pole.)
As a result, “you get an alternating magnetic field that forces the electron beam to deviate left and right,” explains Daniel West. He’s a former biology teacher who now gives tours at Argonne National Laboratory. “Any time you do that to a high-energy beam of any sort, it’s going to [lose] some energy.” He compares the effect to that of a bus swerving back and forth at high speed. If you were inside the bus, you would feel the energy from its movement pushing you from side to side.
In this case, the high speed of the electron beam gives it lots of energy. It throws off that energy as it wiggles. As a result of the beam’s speed, the energy released is in the form of photons known as X-rays. These X-rays have short wavelengths of 0.01 to 10 billionths of a meter. The photons shoot off in bunches, or pulses, at rapid-fire speed.
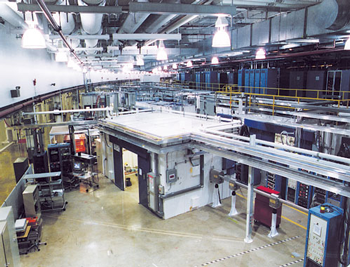
The shape of the ring and the placement of the steering magnets means the rink can emit up to 70 of those X-ray beams, West says. Equipment directs some of those beams to more than one place. So there are more than 90 possible stations for experiments.
Remember how magnets bend the electron beam so that it curves into a circle? The emitted X-rays don’t do that. With nothing to curve the exiting beams, West explains, “the X-rays keep going straight.”
Depending on the work, equipment at these stations can filter or “tune” the X-rays to certain wavelengths, or energy levels. Different wavelengths work better for different types of experiments. Stations also can be set up to work with different types of materials. Some researchers work with metals or ceramics, for example. Other researchers may want to study proteins produced by living cells.
At some stations, the X-rays will bounce off the surface of a sample. “The way they are reflected can reveal exactly what kind of atom is present at each point on [the sample’s] surface,” West says, and precisely how far apart they are.
At other stations, X-rays will pass through a sample. As they do, they scatter or spread out. As these X-ray photons come out the other side, they produce different patterns. This gives clues to the arrangement of atoms within the sample. Computers analyze the X-ray exit patterns. With those data, scientists can measure energy loss from atoms in a sample. Such knowledge can help scientists and engineers make materials that last longer, work better or have new uses.
Story continues below video.
Using the beam
The Advanced Photon Source is a U.S. government research lab. But scientists and engineers come from all over the world to use it. For most of the year, its stations are used around the clock, six days a week.
“A huge part of my job is to set these experiments up for success,” Upton says. Seeing the first results from a sample sometimes feels “really magical,” she adds. “You never know if it’s going to work.”
A lot of her work deals with energy and electronics. For example, one project showed how a crystal material that contained nickel changed its electrical properties at different temperatures. Scientists knew that at different temperatures, the material could either act like a metal, conducting electricity, or become an insulator. What they didn’t know was why.
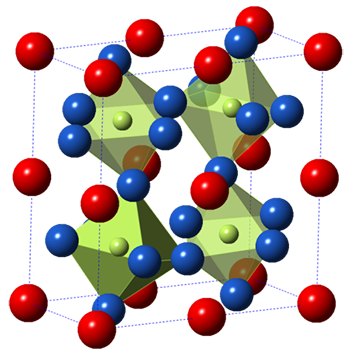
Upton’s team used three X-ray-beam stations to compare a material’s properties at different temperatures. Equipment at each station gave different measurements about the material. Think of it, she says, as “a bottle of liquid and you want to know what it is. You can use your eyes to tell the color and your nose to determine the smell,” Upton says.
In this case, one station might measure a material’s structure very accurately. Another might give information about what was going on with a nickel atom’s electrons as they moved to different energy levels. (Electrons orbiting an atom may jump up or down based on their energies.)
In fact, Upton found, at low temperatures the crystal had slightly fewer electrons on its nickel atoms. Meanwhile, when colder, the material’s atoms of neodymium (another metallic element) showed a small increase in how many electrons they hosted.
“These two measurements make researchers think that electrons are moving from the nickel atoms to the neodymium atoms as the crystal changes from a [conductor] to an insulator,” Upton says. Scientists hadn’t seen such a shift by electrons before.
Why do they care about this? The more scientists understand about what goes on inside a material — such as this crystal — the more likely they are to discover new uses that depend on these properties.
Explains Upton: “The goal of this type of experiment is to be able to design custom electronic components.” For instance, a device might need a part that will conduct electricity well at 21° Celsius (70° Fahrenheit) and even better at 27 °C (80 °F).
Roadmap to a vaccine
Erica Ollmann Saphire is a molecular biologist at the Scripps Research Institute in La Jolla, Calif. She used the Advanced Photon Source to learn more about the virus responsible for Lassa fever. Its victims develop breathing problems, stomachaches, vomiting, diarrhea, heart problems, seizures and more. They also can bleed from the gums, nose, eyes and other body parts. Each year, this nasty infection sickens some 300,000 people, mostly in West Africa.
People tend to pick up the potentially lethal virus after making contact with infected rats or with bodily fluids from sick people. Pregnant women are especially likely to die from Lassa fever. Of those who survive, many end up deaf.
“There are no vaccines or treatments yet available for Lassa virus,” Saphire says. To help change that, her team conducted research using the Advanced Photon Source. Specifically, they probed the germ’s means of attack.
The virus attaches to and invades new host cells using a protein on its surface called GP. Patients who survive the disease will have antibodies to that protein. Scientists want to now create a vaccine that would trigger the body to make those same antibodies. Then it could prevent new cases of the disease.
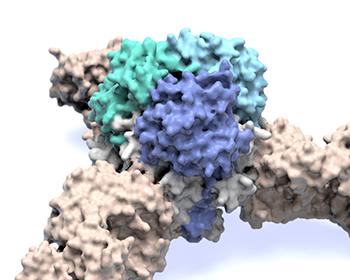
But no one knew what the protein looked like. So they didn’t know how to make a vaccine to target it. “Without the structure of GP, they were hunting blindly,” Saphire explains.
The GP protein usually wants to fall apart and change shape, she explains. So her group spent 10 years trying to stabilize this protein so that wouldn’t happen. Researchers in Africa helped with that effort. Eventually, the team brought their stable protein to the Advanced Photon Source. It let them see, for the first time, this molecule’s tripod-like structure.
That structure is now “the roadmap to make a vaccine against Lassa virus,” Saphire says. Similar techniques might help work toward targeting proteins in other viruses as well. Her group shared its findings in the June 2, 2017 issue of Science.
A brighter future
Argonne National Laboratory is currently planning a $770 million upgrade of the Advanced Photon Source. This will make its X-rays brighter, somewhat “like switching from a 60-watt to a 120-watt bulb,” Upton explains. Other improvements will bring the X-rays more in sync with each other, giving it a sharper focus. That will allow it to peer into smaller samples.
Work on the upgrade could be finished as early as 2023. One day, perhaps you or your classmates might even work on a project there. “If they like math and they like tinkering, they should join us!” Upton says.
Reporting for this piece was made possible by an MBL Logan Science Journalism Fellowship in Chicago. It was arranged by the Marine Biological Laboratory in Woods Hole, Mass., and the University of Chicago.