The exotic ‘atom’ positronium surprises scientists
Measurements of it don’t match careful predictions, which is confounding physicists
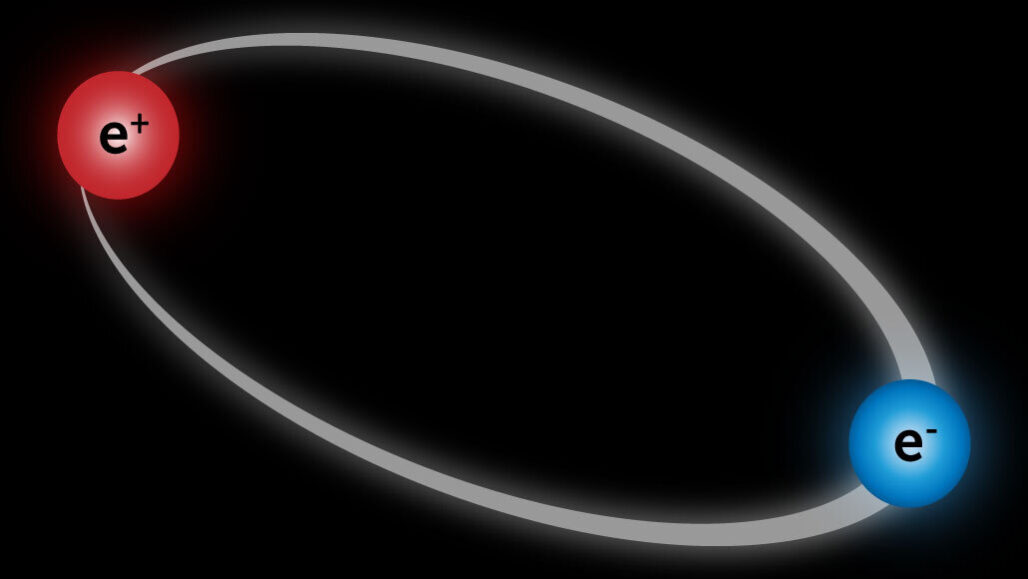
This is the concept of the exotic “atom” positronium, which has been known about for decades. It consists of a negatively charged electron (blue) and a positively charged positron (red). A new study of it disagrees with predictions, and scientists can’t figure out why.
T. Tibbitts
Positronium is positively puzzling.
This exotic “atom” has no nucleus. As such, it should be simple to understand. Yet a new measurement of it does not match what scientists had predicted. Physicists are now at a loss to explain why that might be.
The riddle was reported August 14 in Physical Review Letters.
A flaw in earlier calculations seems unlikely, the researchers say. So does any mistake in how the new measurement was made. And unknown phenomena — such as undiscovered particles — also don’t seem likely, adds Jesús Pérez Ríos. He’s a theoretical physicist who did not take part in the new study. He works at the Fritz Haber Institute. It’s part the Max Planck Society in Berlin, Germany. So what is the explanation? “Right now,” he says, “the best I can tell you is, we don’t know.”
Positronium is made of matter and antimatter. Electrons and other familiar basic particles of matter have less common counterparts. Those counterpart particles are known as antimatter. The antimatter particle’s electric charge is the opposite of its matter kin. Positronium is composed of electron and its antiparticle, a positron. Electrons are negatively charged. Those positrons are positively charged.
Positronium’s electron circles in an orbit with a positron. The two are attracted by their opposite charges. It’s how electrons are attracted to the positively charged nucleus in most atoms. Except positronium doesn’t have a nucleus.
For more than 60 years, scientists have been able to create positronium. But the nucleus-free atoms don’t last long. Their partnerships are unstable. They enjoy just a brief dance of death lasting perhaps hundreds of nanoseconds. The reason: Matter and antimatter annihilate each other on meeting. And, eventually, that’s what happens to positronium.
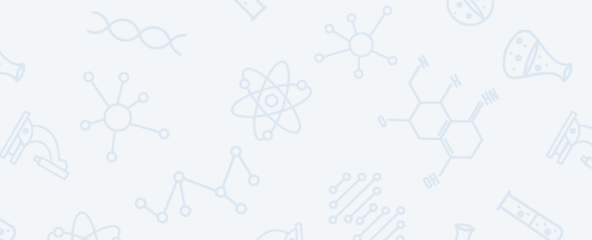
Educators and Parents, Sign Up for The Cheat Sheet
Weekly updates to help you use Science News Explores in the learning environment
Thank you for signing up!
There was a problem signing you up.
So simple, yet so challenging
This atom’s structure is appealingly simple. That should make it easy to precisely test quantum electrodynamics. That’s a long name for the theory that explains how electrically charged particles interact.
Physicists in England at University College London measured what’s known as the fine structure of these atoms. Positronium can exist in one of several states. These states are known as energy levels. The fine structure is the separation between two specific energy levels in the atom.
To form positronium, scientists first sent a beam of positrons colliding into a target. There, this beam met up with electrons. The researchers altered some of the resulting positronium atoms with a laser: It put them in a particular energy level. Then the team bathed the atoms with microwave radiation. This caused some of them to jump to a new energy level.
The researchers pinpointed the frequency of microwave radiation needed to make the atoms take this leap. That told them the size of the gap between the energy levels.
Their calculations predicted the microwave frequency would be 18,498 megahertz. In fact, they needed a frequency some 0.02 percent higher. That may not sound like much. But the estimated error for such tests was only about 0.003 percent. So the difference was far bigger than expected.
What does this mean?
The team searched for anything that might explain the difference. And they came up with — nothing. More tests are needed to try and understand the mismatch, says Akira Ishida. He’s a particle physicist who was not involved with the study. Ishida works at the University of Tokyo, in Japan. If new measurements don’t shrink the difference, he says, “the situation becomes much more exciting.”
The initial prediction for the energy-level spacing also seems to have been good. In this field, these predictions involve making calculations to a certain level of precision. This requires leaving out some things that are less significant and harder to calculate. The left-out things are expected to be too small to cause any difference. Still, “it’s conceivable that you could be surprised,” says Greg Adkins. He’s a theoretical physicist at Franklin & Marshall College in Lancaster, Pa.
If the new tests and the predictions both seem accurate, then the mismatch might point to some new subatomic particle.
That, however, seems unlikely. A new particle’s effects probably would have shown up in earlier tests. For example, says Pérez Ríos, positronium’s energy levels could be affected by a hypothetical axion-like particle. That’s a lightweight particle that has the potential to explain dark matter. (Dark matter is an invisible type of matter thought to permeate the universe.) But if such a particle was behind the mismatch, researchers should also have seen its effects when measuring the magnetic properties of the electron.
That leaves scientists still searching for an answer, says David Cassidy. A coauthor of the study, he also works at University College London. “It’s going to be something surprising,” he concludes. “I just don’t know what.”