Cool Jobs: A world aglow
Three scientists explore all-natural ways to make light — and their promise for new technologies
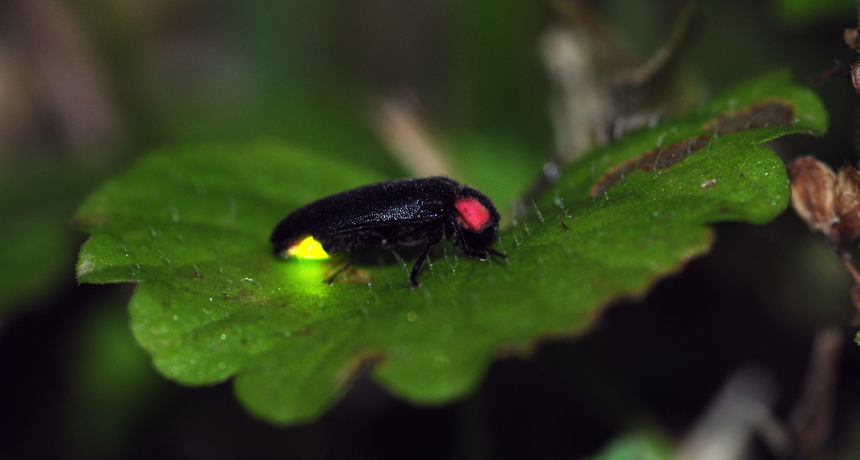
Fireflies, like the one shown here, make their glow chemically. Some animals use other techniques. Scientists are now investigating how we might harness these processes to light the human world.
kororokerokero/istockphoto
Out in the ocean, a see-through squid swims inconspicuously. Sunlight from above shines into the water and through the squid. Well, right through most of it anyway. Only the squid’s eyes block the sunlight, casting a shadow below. That shadow poses a danger for the squid. Deep-sea predators below are looking upward, searching for such shadows. That’s how they find food.
Many species of glass squid, however, have evolved a solution to this problem. They emit light from under their eyes. Now, from below, the animal seemingly disappears. As nonsensical as it might seem, they use light to hide.
And these squid are not alone in harnessing light as a survival skill. Deep below the ocean’s surface is a world of light. Almost every creature down there shines in some way. Consider the sea worm. It shoots out yellow sparks to scare off predators.
Alison Sweeney is a physicist at the University of Pennsylvania in Philadelphia. “In the deep ocean, bioluminescence is the rule, rather than the exception,” she notes. By bioluminescence (BY-oh-loom-in-ESS-ens), she’s referring to the ability of many organisms to give off light.
Hiding from predators is far from the only reason animals generate light. The viperfish, for instance, emits a blinking beacon that lures prey — to become its dinner. Even that emblem of summer, the firefly, emits light. It’s goal is to find a mate. The pulsing yellowish green flashes not only tell potential mates “I’m here,” but also warn predators that this insect is too toxic to make a healthy lunch.
Long ago, people figured out how illuminate their world with electric lighting. But people are far from the only creatures that can light the darkness around them. Many animals do too. And as strange as it sounds, even bubbles may sometimes emit light.
Here we meet three scientists who study such all-natural sources of light. What they’ve been turning up may lead to better devices for illuminating the human world. Their findings might even yield better sources of energy — and perhaps a better understanding of geriatric stars lurking in deep space.
Story continues below image
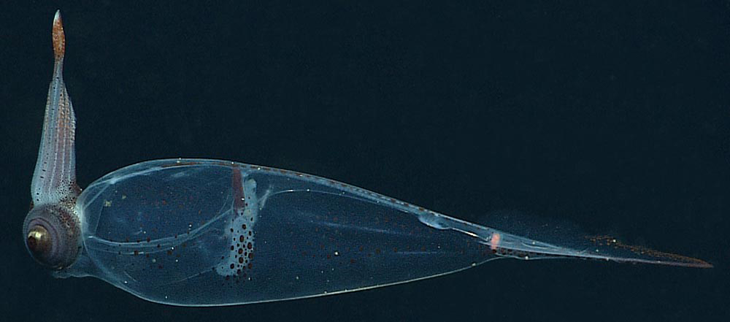
The chemistry of light
Many animals that make light have special cells where chemical reactions create a glow. Glass squid, for example, have patches of these cells around their eyes. The light they issue will now fill in the shadow their eyes would have otherwise cast. A firefly, in contrast, has a clump of these cells in the last segment of its abdomen. They all glow at once to make a bright flash.
Where those cells are and how closely they are spaced will depend on what the animals do with their light. The variety is a bit “like all the different types of lights you find at Home Depot,” Sweeney says. “You have huge flood lights, Christmas lights and flashlights.” Each has its purpose.
Sweeney became interested in the ability of animals to generate light when she was in college. How sunlight affects plants and animals fascinated her. Eventually, she decided to probe how this connection evolved.
In graduate school, she began to study how animals emit and use light. That’s what led her to study a genus of glass squid, called Galiteuthis (Gal-ih-TU-thiss). Like fireflies, the glass squid relies on a trio of chemicals to make its light. One is the element oxygen. Another is a pigment called luciferin (Loo-SIFF-ur-in). The last is an enzyme (a molecule that speeds up a chemical reaction) called luciferase (Loo-SIF-ur-ace). When luciferin and oxygen interact, they produce light. Luciferase speeds up that reaction. (Both luciferin and luciferase take their names from the Latin word lucifer, for “bearing light.”)
The glass squid cells that emit light look like thin cables, each about 50 micrometers long. That’s about the width of a human hair. Sweeney thought the cells also acted like cables. They seemed to direct their light downward, toward the ocean floor.
But while studying these cells carefully, she and her colleague Amanda Holt turned up a surprise. Those cells were “leaky,” Sweeney explains. They lost light all along their length. That meant light was even exiting the cell sideways.
At first, this struck the women as inefficient. But then Sweeney considered the animals’ world. The squid would need to hide from predators lurking not only below but also to both sides. So they would have to avoid casting eye shadows to either side. And the leaky light did just that. It effectively hid the squid’s eyes from all angles.
Sweeney and Holt reported their finding in the June 2016 Journal of the Royal Society Interface.
“These animals are masters of manipulating the tools to make light,” Sweeney concludes. They seem to have tricks for working with light in extremely small spaces (a single cell) and in bizarre environments.
Researchers hope to make new devices that do the same thing using nanotechnology. That term refers to things that are made from structures with sizes on the scale of 100 billionths of a meter (some 25 billionths of an inch) or less.
One idea is to use the luciferin-based chemical reaction to make tiny structures glow. These nanorods might offer an alternative to LEDs, or light emitting diodes. As their name suggests, LEDs are electronic devices that emit light. They do so by allowing electrons to pass through a semiconductor. Such a material can transmit electrons, but won’t heat up the way the metal filament does in traditional (incandescent) light bulbs. That heat is just wasted energy. So LEDs are much more efficient than traditional light bulbs at providing a glow.
But LEDs have a problem. They need electricity. Nanorods that glow via a chemical reaction wouldn’t. So this might be one way to make LEDs even more efficient. Still, inefficiencies in generating light aren’t all bad, as Sweeney’s work shows. Glass squid use inefficient ways of generating light to live better lives. “We want to understand how squid do it, so we can do it too,” she says.
Light’s flow
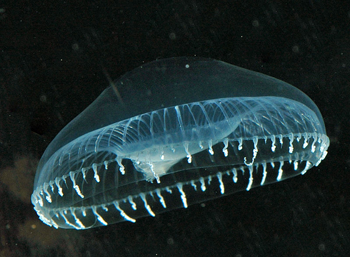
Not all animals rely on chemical reactions to make their glow. Some instead fluoresce (floor-ESS). This process converts one form of light to another.
Certain molecules in their cells absorb light of one wavelength, or color. This bonus energy excites the molecules. But they don’t want to stay excited. So they release this energy — but at another wavelength, usually a longer one.
For example, an object that absorbs ultraviolet radiation may re-emit that energy as visible light. And that will make it glow.
Matt Liptak got interested in studying molecules that fluoresce after one of his colleagues hit a snag in his research. Liptak is a chemist at the University of Vermont in Burlington. His colleague Ivan Aprahamian is a chemist at Dartmouth University in Hanover, N.H.
Aprahamian was studying molecules made from three materials: the elements boron and fluorine, and a compound called hydrazone. He was interested in these molecules because they are molecular rotors — tiny machines. In them, two parts of the molecule can rotate with respect to one another.
The molecules that interested him would fluoresce after violet light shined on them. This had been expected. What was not expected: In a thin, runny liquid, such as water, the molecules glowed weakly and red. In a thicker liquid, such as maple syrup, they instead glowed bright green.
Story continues below video
Courtesy of Morgan Cousins and Matt Liptak, UVM
That wasn’t supposed to happen. In fact, in the thicker materials the molecules shouldn’t glow at all!
Fluorescent molecules typically absorb light, which then makes them vibrate, stretch and give off light. All of these processes release energy, which brings the molecules to a more “relaxed” state. In that relaxed state, the molecule will hold less energy. So as the molecule relaxes, it can shed extra energy as light.
Yet the molecules Aprahamian studied glowed more strongly when they were put into thicker and thicker liquids. This was unexpected since most molecules that fluoresce emit less light in thicker liquids. Clearly, something odd was happening.
Aprahamian asked Liptak for help in figuring out why his molecules didn’t relax in the thick liquids. Liptak’s team made computer models of the fluorescent molecules. (These are analyses done with a computer to mimic how a physical system works.) This model by Liptak’s group led to predictions about the molecules. The researchers could then test those predictions in experiments. And when they did, they found that these molecules behaved very differently from most fluorescent compounds.
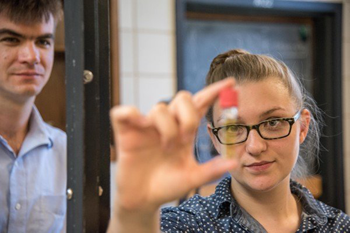
“The thickness of the fluid, what we call its viscosity, was shutting down the molecule’s relaxation,” Liptak realized. This didn’t let the molecules vibrate well. So they could not twist at the bottom. That kept them from shedding as much heat as might have been expected. The “excited” molecules responded by emitting light, which led to a brighter glow.
In essence, the research team had discovered a new way fluorescence can work. They described it in the September 2016 Nature Chemistry.
“It’s really exciting,” Liptak says, “because we realize now there’s a whole world of these molecules to explore.”
If the team can find molecules that emit light like these molecules, but even more brightly, then these chemicals might also find use in helping improve LEDs. No one knows, yet, the potential that such molecules might hold for the next generation of LEDs. But they “open up a new research pathway,” Liptak says.
Bright bubbles
Bubbles don’t seem like they should be able to emit light. But under the right conditions, they do through a process known as sonoluminescence (SO-no-loom-in-ESS-ens).
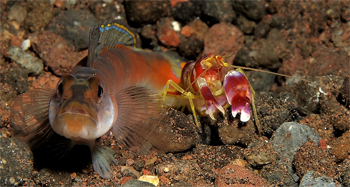
Bubbles are little pockets of gas. Sound waves can crush them. And doing so, the bubbles will release energy in a fantastic burst of heat and light. This happens in nature, when snapping shrimp clamp their claws shut.
As the two parts of the claw snap into each other, the change in pressure makes bubbles shoot out. It also triggers the sound of a snap. That sound travels as a wave. As it does, it causes changes in the pressure and density of the water.
Such large pressure changes can squeeze bubbles, making them collapse. When that happens, the gas inside heats. In fact, that gas can get hotter than the surface of the sun. In may get so hot that electrons are pulled off of their atoms. This creates plasma, a soup of electrons and charged atoms.
Eventually the electrons and atoms recombine. Some scientists think that’s when the burst of light emerges. All of this happens in less than a billionth of a second.
It’s pretty incredible, notes Alex Bataller. He is a physicist at North Carolina State University in Raleigh. And there’s no reason this way of making light should be restricted to snapping shrimp. He says that people could use it, in theory, to make energy.
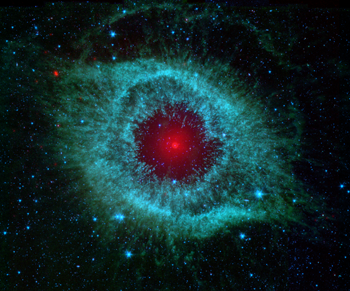
We use a lot of energy to power our gadgets, heat our homes, drive places, keep the lights on, cook food and do all of our other daily tasks. Generating that energy causes pollution. And common sources of energy, such as coal and oil, won’t last forever. So scientists are looking for cleaner, sustainable ways to make energy.
One way would be to fuse atoms together. Fusion releases a lot of energy. It’s the process that fuels the sun’s production of heat and light. The sun’s energy will last for another 5 billion years or so. Scientists would like to figure out how to safely fuse atoms to generate energy on Earth.
Sonoluminescence might be the ticket. But scientists need more control over the process than comes from the bubbles and light that snapping shrimp make. Scientists also need to know more about what’s happening inside the bubbles. What’s the temperature? What’s the pressure? Can you make the gas inside the bubbles get hotter than it normally would? Can you get it hot enough to fuse atoms?
These are questions Bataller has tried to answer. Until recently, he was a graduate student at the University of California, Los Angeles. There, he spent a lot of time shooting laser beams into tiny bubbles. These bubbles were micrometers in size. That’s just a few ten-thousandths of an inch — about the size of a single red blood cell.
He focused the laser to about the same size. The goal was to get the bubble to absorb the laser light. That should boost the temperature of the gas inside.
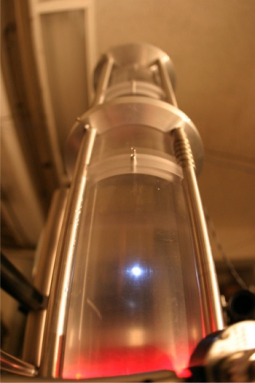
Ultimately, Bataller wanted to get the bubble’s internal temperature to reach some 10 million degrees Celsius (18 million degrees Fahrenheit). Then, when the bubble collapsed, maybe — just maybe — the gas inside would get hot enough to fuse its atoms.
So far, no one has gotten this bubble fusion to work. It’s unclear if there’s any way to get the inside of a bubble that hot. But researchers are continuing to try.
Bataller, however, has moved on. He is now using what he learned blasting bubbles with lasers to study other materials. For instance, he’s probing semiconductors and the plasmas that can form inside them. This work, still in its early stages, also has to do with creating cleaner energy.
And even if bubble fusion doesn’t work, Bataller says, scientists can still use sonoluminescence to study a type of dying stars known as white dwarfs. They are about as hefty as the sun but only the size of Earth. Their cores have collapsed. In the process, they’ve blown off a lot of gas. But they still contain plasma, like light-emitting bubbles do. So studying those bubbles may offer insights into white dwarf stars.
As the work of these researchers shows, science and engineering have much to learn from how nature can set bits of the world aglow.
This is one in a series on careers in science, technology, engineering and mathematics made possible with generous support from Arconic Foundation.