Explainer: Winds and where they come from
Here’s an explanation for how and why the wind blows
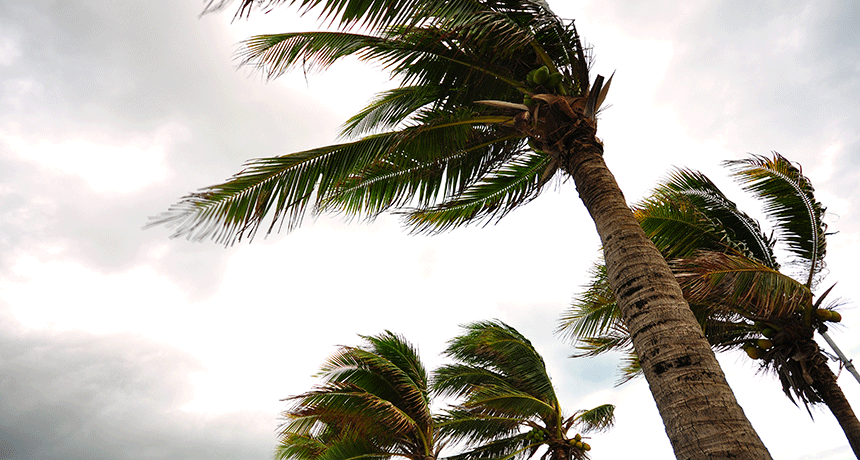
Temperature and pressure are critical factors in the creation of a windy day.
behindlens/iStockphoto
Hear that flag snapping sharply against the flagpole? See those kites flying high overhead? Feel that cooling breeze coming in off of the water?
Wind is all around us. It arrives in many shapes and forms. Wind may be an elegant mood-setter or a furious early warning of a dangerous storm. Although few people give much thought to wind — unless it’s threatening — those rivers of moving air drive the weather in ways that rule our environment.
There are many different types of wind. Each forms in different ways. But essential to all are changes in air pressure.
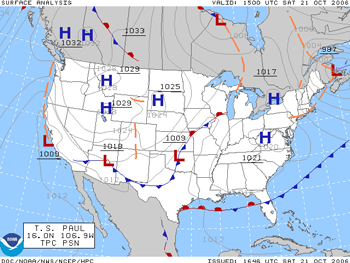
TV weather forecasters regularly point on maps to areas of high and low pressure. And that makes sense because changes in air pressure are what lead to wind — the flow of air. In fact, wind is Mother Nature’s way of equalizing differences in air pressure.
Air pressure is the force that air exerts toward whatever contains it. The pressure of air in a balloon is higher than that of the air outside. That’s why most of the air will leave a balloon whenever it gets a hole. When it comes to the atmosphere, air pressure describes the weight of air over a given site. It is determined by that parcel of air’s temperature, volume and density.
Expanding air produces regions of “high pressure.” These push nearby air away. Contracting air creates zones of “low pressure.” They pull nearby air inward. That’s why the wind blows: It moves from regions of high pressure to those where pressure is lower. The zone between the high- and low-pressure areas is known as a pressure gradient, or a zone over which the pressure varies from high to low.
Thermal wind balance
Thermal wind is the first of four main types of atmospheric flow. The most complex type of wind, it drives weather systems across the globe. It’s born from differences in the temperatures between the equator and the poles.
Picture a column of air from the ground to the top of the troposphere (TRO-puhs-sfeer) — that layer of atmosphere in which we live. As the sun beats down on it, this air heats up and expands. That makes the top of the column rise. This is common near the equator. If a column of air cools, such as at the poles, it contracts and shrinks. That same stack of air — still weighing the same amount — will now be shorter and denser.
This means that imaginary surfaces of constant density slope down toward the poles. That slope isn’t constant. These lines rise up and down like bumps and wrinkles in a blanket, depending on local conditions. But the general downward slope allows masses of air to slide toward the poles.
Thermal wind is what is created as those masses flow down this slope, carrying heat away from the equator. Meteorologists refer to this natural movement of solar energy out of the equator as “poleward heat transport.” Without it, most folks living outside the tropics would be buried beneath a sheet of ice. The equator would also be hot as a furnace.
As sun-warmed air rises near the equator and begins to move toward the poles, it also starts to drift eastward. This is due to Earth’s spin. It swirls the air from west to east around the planet.
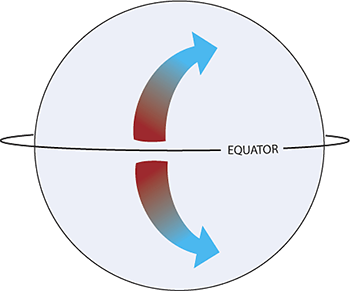
That poleward-moving air also speeds up — dramatically. This is because Earth is an oblique (Oh-BLEEK) spheroid. If you took horizontal slices of the planet, those slices would be widest at the equator and narrowest at the poles. As Earth’s radius “shrinks” as one approaches the poles, the air has to speed up. This is because the air gets funneled into a smaller and smaller path. As it does so, its flow rate increases. (This process is due to what’s known as the conservation of angular momentum.) In the Northern Hemisphere, this makes the air flow to the right with increasing speed. This swirling action is known as the Coriolis force.
Earth’s rotation and the change in the planet’s radius mean that moving air will always want to turn a bit to the right in the Northern Hemisphere (and the opposite direction in the Southern Hemisphere). This affects everything. A football tossed from one end of a stadium to another will naturally deflect 1.26 centimeters (a half inch) to the right! It’s also why winds in the upper atmosphere are relatively weak near the equator. Closer to the mid-latitudes, they howl. They’ve curved so much to the right that they often are speeding eastward at an impressive clip.
The jet stream
This is how the jet stream forms. This current of air snakes around the planet at speeds greater than 322 kilometers (200 miles) per hour. It’s found winding its way directly overhead of the strongest temperature contrasts at the surface.
This temperature gradient creates a steep density “hill” in the atmosphere where the air quickly sloshes down. The more rapidly it moves, the more the northern jet stream curves east. It’s just like riding a bicycle down a hill: The steeper the slope, the faster you go.
But as the air moves poleward, it never actually gets to the poles. Instead, it curves to the right rapidly because of Earth’s rotation and that Coriolis force. As a result, the jet stream meanders as it circles the Earth in each hemisphere. In the North, it moves air west to east in a circle around the mid-latitudes (and the opposite in the Southern Hemisphere), changing its path from season to season.
Poleward of the jet stream, the atmosphere is turbulent. Dozens of “eddies” of high and low pressure rotate around the globe, dragging wacky weather with them. On the equator side, the flow is described as “laminar.” That means it’s relaxed, and not chaotic.
Along this temperature boundary, a fierce atmospheric battleground develops. Colliding air masses of different temperatures spin up cyclones and other severe weather. Indeed, that’s why meteorologists refer to the jet stream’s position as a “storm track.”
The position of the jet stream influences the type of weather a region encounters. Consider the Northern Hemisphere, for instance. From December through February, the sun doesn’t reach the North Pole. This allows an extensive dome of super-cold air to bank up nearby. Atmospheric scientists refer to this flowing pool of cold air and low pressure as the polar vortex. It swells in size during winter. And when this flow of cold air surges southward, it pushes the jet stream into southern Canada and the northern United States. That can bring seemingly endless snowstorms to the upper Midwest and Northeast during the dead of winter.
Geostrophic winds
In summer, the poles warm. This weakens the temperature gradient between these zones and the equator. The jet stream responds by retreating some 1,600 kilometers (a thousand miles) northward. Now, the weather in the lower 48 U.S. states calms down. Sure, scattered thunderstorms erupt from time to time. But there are no huge storm systems spanning 1,600 kilometers or more to influence day-to-day events. Instead, the weather becomes geostrophic (GEE-oh-STRO-fik) — meaning relatively tranquil.
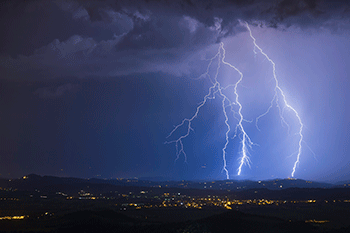
Ordinarily, air would flow from high pressure to low pressure. It would move across a pressure gradient. So the driving force would be known as the pressure gradient force. But the Coriolis force is still at play. So as parcels of air try to move down the gradient, they’re tugged to the right in the Northern Hemisphere (and the opposite direction in the southern one). These two forces cancel out. Like a perfectly-matched game of tug-of-war, the air isn’t yanked in either direction. It just meanders slowly around large pressure systems.
As a result, the air ends up circling around high- or low-pressure systems without moving toward or away from them. Closer to the surface, the flow is slightly ageostrophic (meaning the winds are no longer in complete balance), due to the effects of friction with things at or near the surface.
Other large-scale wind-balancing effects
Sometimes, however, a low-pressure system spins so fast that a third force develops. It’s the same outward shove you feel on a merry-go-round or a vehicle rounding a corner. This is centrifugal force.
Rings of air in constant balance between these two forces spin around a storm’s center indefinitely. Their rather constant distance from the center is due to what’s known as cyclostrophic (Sy-klo-STROW-fik) balance. This represents a harmony — complementary actions — of the pressure-gradient and centrifugal forces.
On rare occasions, the Coriolis, centrifugal and pressure-gradient forces can all counteract one another. This perfect trifecta marks what scientists call gradient wind balance. It’s not worth a lot of fanfare. It does, however, dictate which way air parcels will move along the outer edges of a cyclone, any spinning column of air.
Clearly, there are a lot of moving parts that control the way the wind blows.
Local winds
The last category of winds are the ones you experience every day. And they’re different depending on where you are. Head down to the beach, for instance. On sunny days in the afternoon, air over land warms and rises. Cooler air sitting above the ocean rushes in to coastal regions, filling the void caused by the air rising over land.
This generates a line of puffy little cumulus (KEWM-u-lus) clouds that die out after the sun sets. Along peninsulas like Florida, colliding sea breezes can result in convergent winds. These colliding air masses force pockets of moist air high up into the atmosphere, forming thunderstorms. That’s why folks in the Southeast always carry umbrellas, even on sunny mornings. The “self-destruct” sunshine routinely generates scattered afternoon boomers.
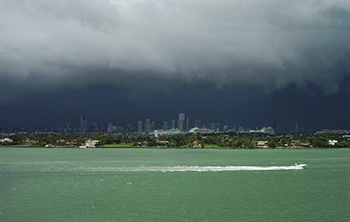
The same process that sparks these storms reverses overnight. Since the ground cools faster than the water, the direction of the flow of air reverses. Instead of a sea breeze, a “land breeze” develops. Now, storms move out from the land, to the ocean. That’s the reason many people along the Gulf Coast can enjoy gorgeous offshore displays of evening lightning.
Wind also can vary locally along stationary fronts. These are the very sharp boundaries between regions of warm and cold air. Sometimes, stationary fronts can become hung up in valleys. When they do, the warm and cold air masses — winds — can slosh back and forth. Like water and oil in a bowl, they don’t mix. Instead, they just push each other back and forth like angry ocean waves. This can trigger dramatic temperature swings within short periods of time.
One particularly noteworthy example came from the Black Hills of South Dakota on January 22, 1943. A stationary front had established itself along the foothills in the western part of the state. According to the local National Weather Service office in Rapid City, the temperature skyrocketed from -20° Celsius (-4° Fahrenheit) at 7:32 a.m. to 7.2 °C (45 °F) just two minutes later. That afternoon, as the front retreated, over a span of just 27 minutes the temperature plummeted 32.2 degrees C (58 degrees F).
Similar wild swings in the mercury were noted across that region throughout the afternoon. Motorists reportedly had trouble driving because their windshields would fog over — or even crack — when crossing between warm and cold pockets. (Imagine trying to dress for the weather that day.)
Regardless of where you are or what season it is, the wind holds a lot of information. Its direction, temperature and speed all offer valuable clues about the state of the atmosphere. Next time you’re outside, take a second to pay attention to Mother Nature. There’s a lot she has to tell you if you note what’s blowin’ in the wind.
EarthDirect/NASA