Cool Jobs: Big future for super small science
Scientists use nanotubes to overcome mechanical, environmental and optical obstacles
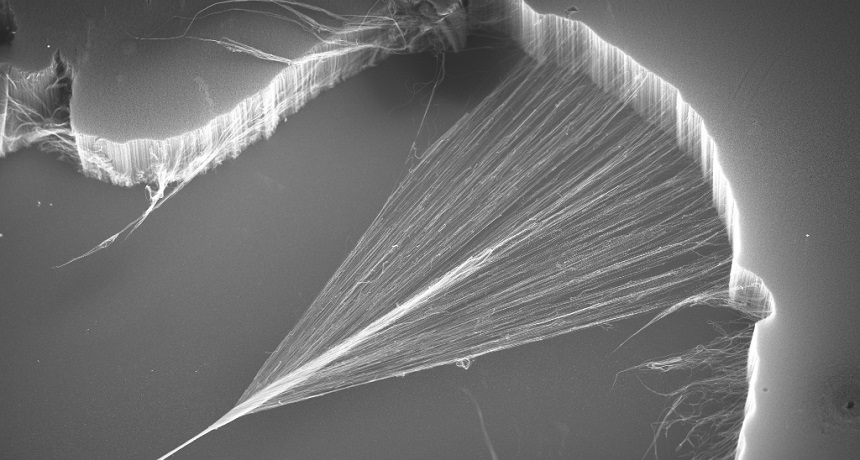
A forest of carbon nanotubes (top and right) is pulled into a cobweb-like sheet, then twisted into a yarn (bottom left).
Ray Baughman/University of Texas at Dallas
A special coating for spacecraft that is blacker than even the inky darkness of space. Artificial muscles powering minuscule motors. Ultra-tiny sponges sopping up major oil spills. These are just some of the up-and-coming uses for exceptionally small structures assembled from individual carbon atoms. While it may sound like the stuff of science fiction, it’s actually science fact — or will be soon.
Welcome to the new frontier in engineering: nanotechnology.
Nanotechnology deals with materials on an extremely small scale. Nanomaterials can include particles, films, wires or even complete structures, including balls and tubes. What’s key is their size. The prefix nano means a billionth. The largest objects created by pioneering nanotechnologists are only 100 nanometers, or 100 billionths of a meter, across. Most are much smaller. Some are only a few atoms — a single nanometer — in diameter. For comparison, a human hair is about 20,000 nanometers (0.0008 inch) wide.
And size is important. As materials shrink, their physical and chemical properties can change. For example, at the nanoscale gold isn’t yellow. Instead it appears red, green, blue or even purple, depending on the size of the particles. This is due to effects that take place on a quantum scale.
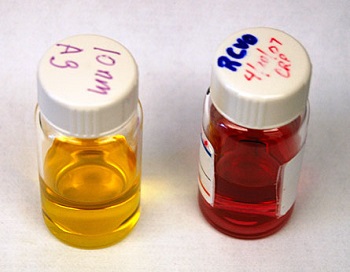
Scientists working with nanotechnology are finding all kinds of ways to put such unusual properties to good use. This story highlights a few of those pioneers who are using carbon nanotubes. The tiny, hollow cylinders that they work with typically have walls just a single atom thick. It is tiny technology — but with an enormous and growing number of potential uses.
Blacker than black
At Surrey NanoSystems in London, England, Ben Jensen grows nanotubes, almost like someone might grow grass from seed. First, he adds a layer of some catalyst to the surface on which he wants to grow the tubes. That might be a sheet of stainless steel or a piece of silicon. The catalysts are nanoparticles of iron, cobalt or nickel. The sheet is then placed inside a vacuum chamber and the air sucked out. Next, the chamber typically is heated to between 750º and 900º Celsius (1,382º to 1,652º Fahrenheit).
A stream of gases is allowed into the chamber. Methane is typically one of those gases. It has one carbon atom surrounded by four hydrogen atoms. Together, heat and the catalyst work to break up the methane molecule, freeing its single carbon atom. That atom then moves to the edge of the catalyst. There, it binds to other carbon atoms. They begin to form a ring around the rim of the catalyst. The catalyst continues to break apart new methane molecules. As more carbon atoms are added, they push the older ones away from the catalyst. Gradually, that ring of atoms grows and takes on the shape of a tube.
Jensen and his team set out to make nanotubes that could be used as a special coating for the outer surfaces of spacecraft. Their goal: to reduce how much light can reflect off of that surface and onto sensitive equipment.
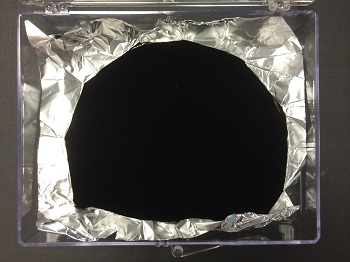
One problem with the standard method of growing nanotubes is the high temperature required. It limits the types of substances on which the nanotubes can be grown. Silicon can withstand those high temps, but it’s fragile. Stainless steel is strong, but it’s also bulky and heavy. And neither is well suited for space travel.
After several years and the development of a new technique, Jensen’s team hit the jackpot. They can now grow nanotubes at a mere 400 ºC (752 ºF). Called Vantablack, their new material meets all of the criteria for use in space. This carpet of nanotubes absorbs 99.96 percent of light, making it the blackest substance known to science. That’s thanks to the arrangement of the nanotubes, explains Jensen.
Photons (packets of light) get trapped in the tubes. There, the light eventually is absorbed by the carbon atoms. Once absorbed, the photons can’t leave. So Vantablack won’t reflect light. On spacecraft, the coating should absorb any glint of light before it gets a chance to reflect. This would keep stray light from hitting sensitive telescope lenses, which have been designed to capture the weak light coming from objects far off in deep space.
Probably the coolest thing about Vantablack, says Jensen, is how completely it absorbs light. When grown on a wrinkled piece of aluminum foil, the wrinkles vanish under the layer of Vantablack. It looks like a hole, even if you hold it up to your nose. If you touch it, you will still feel the hills and valleys. But without any light bouncing off and reaching your eyes, you’d never know those wrinkles were there.
Minuscule muscles
Mônica Jung De Andrade got excited about nanotechnology while a student in Brazil. “At the sub-100-nanometer scale, a given material has new behavior!” she exclaims. And that opens the doors to a wide variety of applications.
Now at the University of Texas in Dallas, Jung De Andrade spins incredibly thin yarns that can act like nano-muscles.
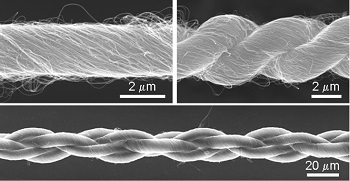
The tubes are grown in what’s called a forest. A normal forest contains stands of trees with their trunks growing close to one another. The nanoforest contains a similarly dense growth of tiny carbon tubes. Jung De Andrade starts by “pulling” that forest of tubes into a “sheet” (see video below). To do this, she swipes one edge of the forest with a small metal tool (a finger works, too). The nanotubes have a lot of surface area. This makes them sticky. So they grab on. When she pulls her hand away from the forest — being careful to pull to the side, not up or down — the nanotubes follow.
As the first row of nanotubes pulls away, they stick to each other. This creates a spider-web-like sheet. The next row of nanotube “trees” follows suit, with each exposed row of nanotubes glomming onto the one behind it. As the sheet stretches, it creates a thread. It almost looks like a sweater unraveling. Jung De Andrade then connects one end of the thread to a rotating motor. As the motor spins, it twists the thread into what nanotechnologists call a yarn.
TINY THREADS Watch scientists create a yarn from a forest of nanotubes. Matt Betts, Oregon State University |
Next, she adds what is called a “guest” material to her yarn. In one experiment, her team sought to create a miniature artificial muscle. It would expand and contract with changes in temperature. To do this, Jung De Andrade chose paraffin wax as the guest material. She placed the wax near the yarn and applied heat. The wax melted. It then oozed through pores in the yarn, filling them with the wax.
Jung De Andrade then twists the yarn even more, over and over, until it coils. Those coils increase the ability of the yarn to contract.
When heated, by exposing it to either a light or an electric current, the wax expands. This fattens the yarn, forcing it to coil more tightly. As a result, the yarn gets shorter. When the heat source is removed, the wax cools rapidly — in a matter of milliseconds. As the wax now shrinks, it untwists. That returns it to its original shape, just as muscles do. The coiled yarns can lift several thousand times their own weight. And they can do so over and over, without wearing out.
MIGHTY MINI Watch a nanotube artificial muscle in action. Matt Betts, Oregon State University |
Such miniature muscles might find use in all types of things, says Jung De Andrade. They might power tiny motors, robots or microvalves. They could find use in ultra-fast camera shutters, sensors or micro-mixers. They might even help make new “smart” textiles. For example, firefighters might wear uniforms made from fabrics woven from nano yarns. The clothes would become less porous when exposed to fire. This would protect them better from the flames.
Tiny sponge, massive clean-up
Mauricio Terrones is looking small to clean up massive messes. This physicist and materials scientist at Pennsylvania State University in University Park has been interested in the very small since he was a kid. (A materials scientist studies how the atomic or molecular structure of a material is related to its overall properties.)
Growing up, Terrones’ favorite movie was “The Fantastic Voyage.” In it, a submarine and its crew are shrunk to microscopic size and then injected into a character’s bloodstream, all to save his life. Terrones marveled at the story. “Is it really possible to get into the body and selectively destroy cancer cells, bacteria and viruses?” he wondered.
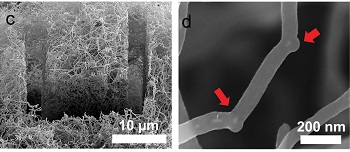
“We then started to notice the sponge behaved very differently when compared to standard carbon nanotubes,” says Terrones. In particular, the sponge absorbed oil from an oil-water mix. Made mostly of carbon, those nanotubes are hydrophobic. That means they repel water. Yet they attract hydrophobic materials. Since oil is hydrophobic, the nanotubes attract it.
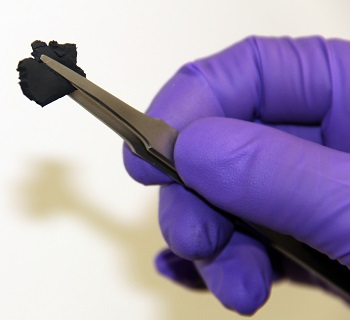
Once filled with oil, the sponges are easy to retrieve. That’s because the iron catalyst used to grow the nanotubes is magnetic. Although there is only a small amount of iron in the sponge, it is enough to allow scientists to move the miniature sponges around using a magnet.
Terrones and his team are working on sponges that can soak up 1,000 times their weight in oil. Terrones imagines a day when he can develop nanotech sponges large enough to sop up even massive oil spills.
“It is important to always dream of things that are impossible now,” Terrones says. Eventually, he believes, “Such science fiction will become a reality.”
Power Words
(for more about Power Words, click here)
atom The basic unit of a chemical element. Atoms are made up of a dense nucleus that contains positively charged protons and neutrally charged neutrons. The nucleus is orbited by a cloud of negatively charged electrons.
atomic Having to do with atoms, the smallest possible unit that makes up a chemical element.
boron The chemical element having the atomic number 5. Its scientific symbol is B.
carbon The chemical element having the atomic number 6. Its scientific symbol is C. Carbonis the physical basis of all life on Earth. Carbon exists freely as graphite and diamond. It is an important part of coal, limestone and petroleum, and is capable of self-bonding, chemically, to form an enormous number of chemically, biologically and commercially important molecules.
catalyst A substance that triggers or speeds up a chemical reaction without itself being affected.
doctoral degree Also known as a PhD or doctorate, these are advanced degrees offered by universities — typically after five or six years of study — for work that creates new knowledge. People qualify to begin this type of graduate study only after having first completed a college degree (a program that typically takes four years of study).
engineering The field of research that uses math and science to solve practical problems.
materials science The study of how the atomic and molecular structure of a material is related to its overall properties. Materials scientists can design new materials or analyze existing ones. Their analyses of a material’s overall properties (such as density, strength and melting point) can help engineers and other researchers select materials that best suited to a new application.
mechanical Having to do with the devices that move, including tools, engines and other machines.
methane A hydrocarbon with the chemical formula CH4 (meaning there are four hydrogen atoms bound to one carbon atom).
molecule An electrically neutral group of atoms that represents the smallest possible amount of a chemical compound. Molecules can be made of single types of atoms or of different types. For example, the oxygen in the air is made of two oxygen atoms (O2), but water is made of two hydrogen atoms and one oxygen atom (H2O).
nano A prefix indicating a billionth. In the metric system of measurements, it’s often used as an abbreviation to refer to objects that are a billionth of a meter long or in diameter.
nanoparticle A small particle with dimensions measured in billionths of a meter.
nanotechnology Science, technology and engineering that deals with things and phenomena at the scale of 100 billionths of a meter or less.
optical An adjective that refers to light or vision.
photon A particle representing the smallest possible amount of light or other electromagnetic radiation.
porous The description of a substance that contains tiny holes through which a liquid or gas can pass.
quantum A term that refers to the smallest amount of anything, especially of energy or subatomic mass.
quantum physics A branch of physics that uses quantum theory to explain or predict how a physical system will operate on the scale of atoms or sub-atomic particles.
sensor A device that picks up information on physical or chemical conditions — such as temperature, barometric pressure, salinity, humidity, pH, light intensity or radiation — and stores or broadcasts that information. Scientists and engineers often rely on sensors to inform them of conditions that may change over time or that exist far from where a researcher can measure them directly.
silicon A nonmetal, semiconducting element used in making electronic circuits. Pure silicon exists in a shiny, dark-gray crystalline form and as a shapeless powder.
subatomic Anything smaller than an atom, which is the smallest bit of matter that has all the properties of whatever chemical element it is (like hydrogen, iron or calcium).
vacuum Space with little or no matter in it. Laboratories or manufacturing plants may use vacuum equipment to pump out air.
valve Something that can reduce or shut off the flow of some gas or liquid through a pipe or other passageway. Some specialized valves may allow a liquid or gas to flow in one direction only.