Nano medicines take aim at big diseases
Minuscule medicines and devices are under development to fight diseases — one cell at a time
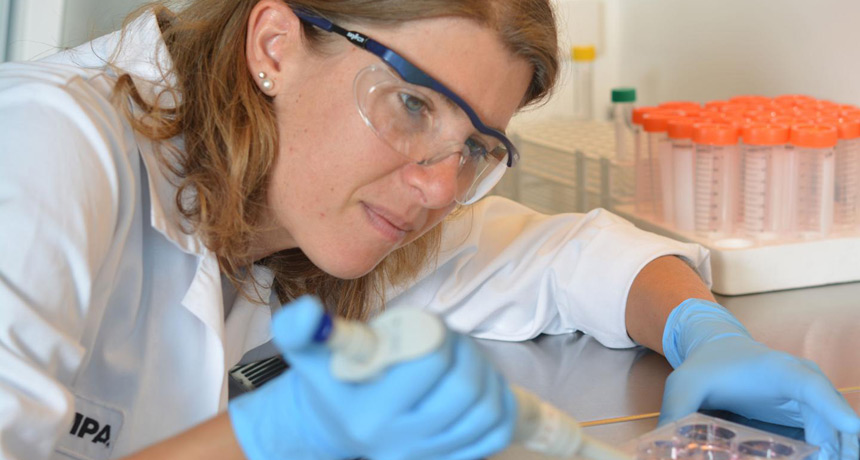
A researcher at the European Nanomedicine Characterization Laboratory in St. Gall, Switzerland, prepares cells for tests on the effectiveness of nanomedicines.
Swiss Fed. Labs for Mats. Sci. and Tech. (EMPA)
The next big thing in medical care could be really, really small. We’re talking nanomedicine. And that nano prefix means these therapies involve things measured on the scale of mere billionths of a meter.
Engineers already use nanotechnology to design better batteries, invisible “inks” and even rewritable paper. In medicine, nano materials could save lives and change the way doctors treat disease.
The teeny tiny particles could travel deep within the body. Some might carry medicines to find and help kill disease. Nanoscale objects might even be part of medical devices placed inside the body.
Nanomedicine is a broad field. “It really covers anything that can be used for care or treatment that’s on the nanoscale,” says physicist James McLaughlan. He works at the School of Electronic and Electrical Engineering at the University of Leeds in the United Kingdom.
New studies show some of the ways smaller can be better in battling disease. Their super-small structures, for instance, can affect how nanoparticles work. Researchers are now trying out tiny tubes, wads, wafers, spheres and other forms. See what’s shaping up in the world of nanomedicine.
Why nano?
Medicines work best when they get to the part of the body where they’re needed. Because they’re tiny, nanomedicines can go where bigger particles can’t.
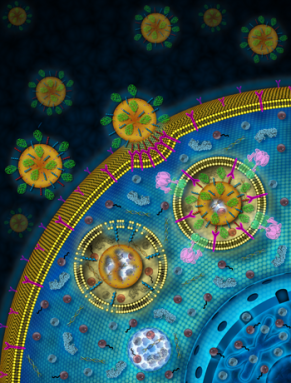
Even medicines as small as one millionth of a meter across may not be able to squeeze through the walls of blood vessels. But much smaller nanomedicines can burrow deep into tissues. Some might even go “straight inside the cells,” McLaughlan notes.
What’s more, size can affect a medicine’s behavior. At the nano scale, the laws of physics get weird. Something called quantum physics starts taking over. Here, at the atomic and molecular level, matter and energy behave as both particles and waves.
“That physics is just as related to our lives, and what goes on in the molecules in our body, as chemistry is,” says Beverly Rzigalinski. She studies the biological actions and effects of medicines at Edward Via College of Osteopathic Medicine in Blacksburg, Va. Importantly, she adds, tiny nanoparticles sometimes act in ways that larger ones can’t.
Rzigalinski works with cerium (SEER-ee-um) oxide crystals. Each particle measures about 10 nanometers across. “One hundred thousand of them would fit in a period at the end of a sentence,” she says.
Cerium oxide has been used in the pollution-control equipment on cars. When added to paints, cerium oxide helps fight rust. In medicine, these nanoparticles might prevent “rust” of a different kind, Rzigalinski says. Namely, harm to brain cells from molecules known as free radicals.
Fighting radicals
A free radical is like the kid in a kindergarten class who can’t keep his hands to himself. Because of its structure, a free radical is anxious to react with other chemicals. Put simply, it will steal an electron from one of its neighbors. In the body, that theft can unleash a type of cell damage known as oxidative stress.
Researchers have linked various diseases to oxidative stress. In Parkinson’s disease, for example, free radicals may contribute to the loss of certain brain cells known as neurons. The affected cells make dopamine (DOE-puh-meen). This chemical has many important jobs. One of its jobs is to help animals to move normally. People with Parkinson’s disease, for instance, often can’t get up from a chair or walk without trouble. Writing and eating also can be hard because of tremors and weak muscles.
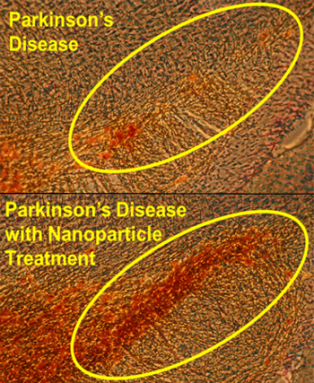
Cerium-oxide nanoparticles can find free radicals and neutralize them. In effect, excess free radicals steal electrons from the particles. Now they are no longer radicals. And that means they no longer pose a threat to healthy cells.
Natural antioxidants, like vitamin C or vitamin E, work in a similar way. They give up an electron to the free radicals. But each of those vitamin molecules can defuse only one free radical. In contrast, cerium-oxide nanoparticles can defuse them over and over.
Rzigalinski’s group thinks the particles use nearby water molecules to recharge — somehow get back any electrons they’ve lost. Once recharged, they’re ready to go back to work. The nanoparticles can defuse “thousands — or hundreds of thousands — of free radicals,” Rzigalinski says.
To see if such particles might work as a medicine, her team gave them to mice. These animals had a form of Parkinson’s disease. One group of mice acted as a control group — meaning they got no treatment. Other groups got a single dose of the particles. Each group got a different dose. The dose that worked best was 0.5 microgram per gram of a mouse’s body weight. It boosted dopamine levels in the brains of mice by almost 50 percent.
“These are extremely low doses,” Rzigalinski stressed. She presented the results in November 2014 at the annual meeting of the Society for Neuroscience in Washington, D.C.
More tests are needed before the treatment is available for people. However, the work might one day help people with Parkinson’s. Rzigalinski is also looking at how the nanoparticles might help treat other conditions, too. Some of her research deals with Alzheimer’s disease, which affects memory. Another project is exploring whether the particles might help with brains injured by a car crash, a sports collision or some other trauma.
Taking shape
A nanomedicine’s shape also can make a difference. The particles Rzigalinski works with look like tiny, crumpled wads of paper. That shape maximizes their surface area. That way, her group’s particles can interact better with free radicals, she says.
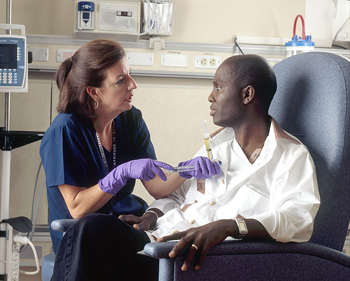
Some other researchers fashion their medicines as tiny spheres. Jae Hyung Park is a chemical engineer at Sungkyunkwan University in Suwon, the Republic of Korea. His group coated nanoparticle spheres of a cancer drug with an artificial material called a polymer. After a while, the polymer will biodegrade, or break down inside the body. In mice, that coating kept the drug from acting until it had a chance to reach the animals’ cancer cells. The team reported its findings last year in Biomacromolecules.
In a similar study, another team of scientists worked with a very toxic cancer medicine. By itself, that medicine would destroy healthy blood-making marrow tissue in mice. But to spare the marrow, these researchers worked with nanoparticles shaped a bit like porous nano-size balls. This design slowed down the drug’s release. Now one dose could kill cancer cells for a longer period of time. At least as importantly, tucking the medicine inside the balls cut down its harm to the marrow.
Scientists at the drug company AstraZeneca in Cheshire, England, described these findings on February 10 in Science Translational Medicine.
Going tubular
Back at the University of Leeds, McLaughlan and his colleagues have used gold nanotubes that look like tiny straws. Their shape might help doctors both find and better treat a cancer, he says.
First those straws have to find the cancer cells. To do this, the researchers coat the tubes with a special chemical. The coating makes them stick to a particular type of cancer cell. Once injected into the blood, the straws will hunt down those cancer cells — and largely ignore all others. Afterward, the researchers will shine near-infrared light onto the outside of the body.
The nanotubes’ shape — the ratio of their length to diameter — makes them absorb energy from that light. Afterward, the nano straws will release that energy again, this time as sound. Ultrasound imaging can listen for that sound and use it to pinpoint where a cancer is hiding. The method worked well to find cancer not only in tissues that were growing in a dish but also in mice.
These same nanotubes might later help with treatment too, McLaughlan says.
Gold is a metal that easily conducts heat. Once doctors find where cancer cells are hiding, they can train a laser beam at that site. This would heat up the nanotubes, essentially frying any cancer cells they had glommed onto. Or doctors might direct heat at the nanotubes as a way to release any medicine that might have been packed inside the nanotubes. Early last year, McLaughlan and his colleagues described tests of this in Advanced Functional Materials.
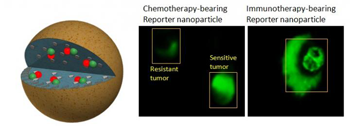
Another type of nanoparticles also would do double duty, but in a very different way.
They look like crumpled wads of paper or wrinkled spheres (think of raisins). Inside them is a drop of medicine containing a special dye. Once they get inside a cancer cell, the particles release their medicine. This kills the cell. Now the dye undergoes a chemical reaction with an enzyme in the cell. When a doctor shines near-infrared light on this tissue, the dye in these dead cells will fluoresce, or glow.
That tell-tale glow confirms the drug had been successful, explains Ashish Kulkarni. He’s a bioengineer on the research team at Harvard University and Brigham and Women’s Hospital in Cambridge, Mass. If the glow doesn’t show up, a doctor would know to try something else, such as a different medicine.
There are more than 100 types of cancer. Each is a different disease with different types of cells. These nanoparticles have worked in lab tests with cancers that initially form in breasts, prostate, lungs, skin and ovaries. Kulkarni and his team described their work this spring in the April 12 Proceedings of the National Academy of Sciences.
Proceeding with caution
Don’t expect your doctor to write a prescription for the nanomedicines described here. At least, not just yet. Some early nanomeds are already helping some patients. But before governments in North America, Europe and elsewhere approve a new medicine’s use, they usually demand rigorous tests to show the treatments are both safe and work well. It takes many steps before a treatment that works in the lab or in animals may be tried in people.
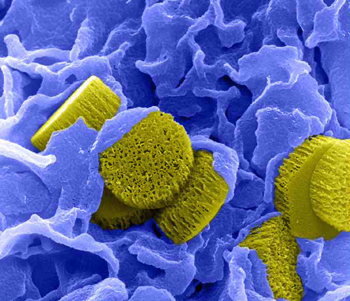
Treatments designed by Rzigalinski, McLaughlan and many other teams must still go through such testing. Along the way, researchers may need to tinker with the design of a drug to limit the risk of side effects.
“Gold itself is not that toxic,” McLaughlan says, talking about his team’s nanotubes. Gold also doesn’t linger long in the body. Still, he says, “It’s going to take a lot more studies” before anyone will try gold nanotubes in people.
And what if they aren’t completely safe? In some cases, doctors will still use medicines, even when they pose some risk. Cadmium is very toxic to the kidneys, for instance. Like some other metals, this one can form toxic free radicals in the body. But nanoparticles with cadmium might boost the potency of radiation therapy for cancer. Patients undergoing radiation treatments often face a high risk of death from their disease. Those patients might be willing to accept some additional risk if there seemed a reasonable chance the cadmium would clobber their killer disease.
So far, Rzigalinski hasn’t found any toxic effects in mice she has treated with cerium oxide. “It doesn’t generate free radicals” in the body, she says. The same physics that lets the nanoparticles work over and over seems to prevent that.
Side effects remain a worry
Yet even with lots of lab tests, scientists might not identify all possible side effects. Some nanomeds may trigger effects “we might not have thought about before,” notes Alan Gaffney. He’s a doctor at New York-Presbyterian/Columbia University Medical Center in New York City. His work there deals with life support for patients during surgery.
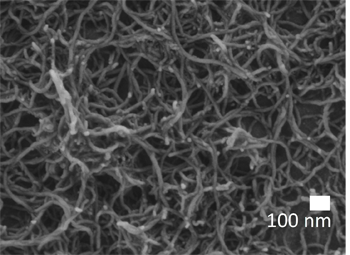
Gaffney recently worked on a project using carbon nanotubes. Carbon usually doesn’t cause inflammation or other health problems, Gaffney notes. For this project, he worked with Marek Radomski and other scientists at Trinity College in Dublin, Ireland. Radomski’s work deals with the health effects of drugs and medical devices.
The challenge is “to engineer the surface of medical devices for them to be safe,” Radomski explains. Ideally, these surfaces should mimic the way the body’s own cells work in the lining of blood vessels, he says. Yet the devices doctors use for heart patients may be made of very unnatural materials.
Take stents. Surgeons insert these net-like devices — often made from metal — into blood vessels to keep them propped open. But blood sometimes sticks to the stents. This could lead to deadly blood clots. Or consider patients put on a heart-bypass machine during surgery. This machine takes over for the heart to pump blood around the body. But its surfaces, too, can also pose a clot threat. The researchers wondered whether covering the inside of such devices with nanotubes might cut that risk of clots.
To test that, they ran human blood through a bypass machine sized for a rabbit. The end result: The nanotube coating upped the risk of clots. This was hardly the result they had hoped for. Radomski’s team reported its findings last year in Nanomedicine.
If that could happen in a machine, perhaps other medical uses for nanoparticles need a closer look too, Gaffney now warns.
Perhaps there’s a way to make those surfaces more like that of a non-stick pan. Another idea: Perhaps designers could cover the surface with teeny tiny dimples, like those on a golf ball. “We are pretty sure that if you want to change the properties of the surface, you’ve got to do something different,” Radomski says.
As in any field of science, knowing what doesn’t work is often helpful. To make nanomedicines work just right may take a lot of trial and error. But one day, such teensy particles might improve medicine in a huge way.