Cosmic timeline: What’s happened since the Big Bang
The biggest changes — and lots of them — took place well within the first second
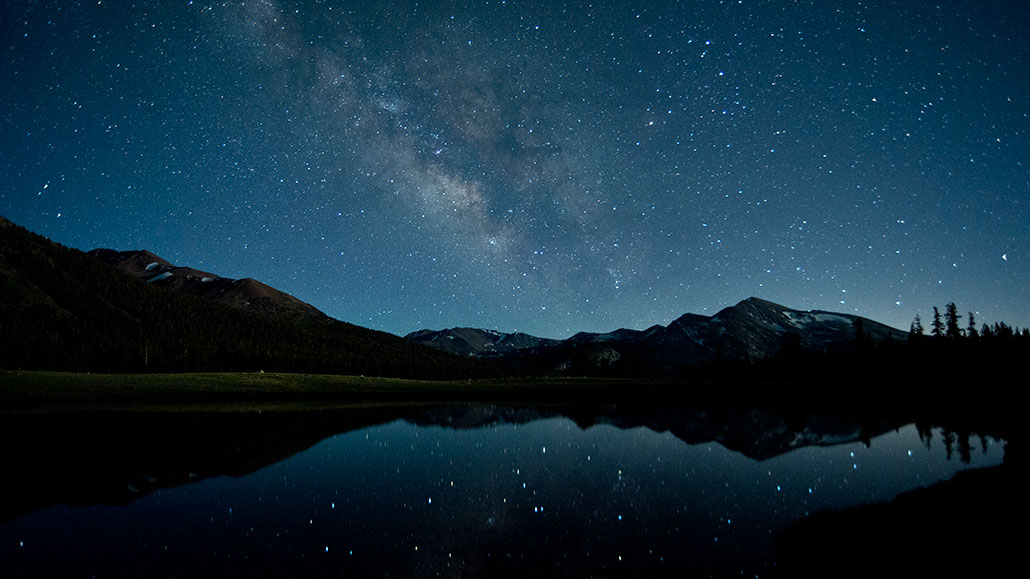
Since the Big Bang, a lot has happened. Our Milky Way is but one galaxy among billions that have evolved over that nearly 14 billion years.
Tony Rowell/Corbis Documentary/Getty Images Plus
By Trisha Muro
When astronomers think about how the universe has evolved, they divide the past into distinct eras. They start with the Big Bang. Each subsequent era spans a different length of time. Important events characterize each period — and lead directly to the next era.
No one truly knows how to describe the Big Bang. We can sort of imagine it as a gigantic explosion. But a typical explosion expands into space. The Big Bang, however, was an explosion of space. Space didn’t exist until the Big Bang. In fact, the Big Bang was not only the beginning of space, it was also the beginning of energy and matter.
Ever since that cataclysmic beginning, the universe has been cooling. Hotter things have more energy. And physicists know that things with very high energy can flip back and forth between existing as matter or as energy. So you can think of this timeline as describing how the universe gradually changed from being pure energy to existing as different mixes of matter and energy.
And all of it began with the Big Bang.
First, a note about numbers: This timeline spans an enormous range of time — literally from the very smallest concept of time to the very largest. Numbers like these take up a lot of space on a line if you keep writing them as strings of zeros. So scientists don’t do that. Their scientific notation relies on expressing numbers as they relate to 10. Written as superscripts, these “powers” — multiples of 10 — are denoted as tiny numbers written to the upper right of a 10. The tiny numbers are called exponents. They identify how many decimal places come before or after the 1. A negative exponent does not mean that the number is negative. It means that the number is a decimal. So, 10-6 is 0.000001 (6 decimal places to get to the 1) and 106 is 1,000,000 (6 decimal places after the 1).
Here’s the timeline for our universe that scientists have laid out. It begins at a fraction of a second after the birth of our cosmos.
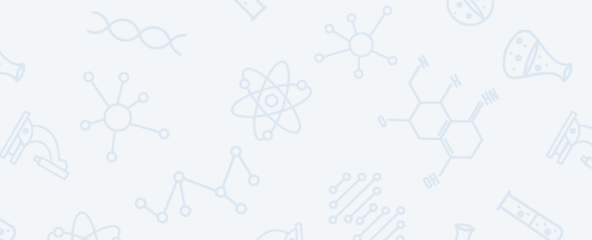
Educators and Parents, Sign Up for The Cheat Sheet
Weekly updates to help you use Science News Explores in the learning environment
Thank you for signing up!
There was a problem signing you up.
0 to 10-43 second (0.0000000000000000000000000000000000000000001 sec) after the Big Bang: This earliest period is known as the Planck Era. It goes from the instant of the Big Bang to this minuscule fraction of a second afterwards. Current physics — our understanding of the basic laws of energy and matter — cannot describe what happened here. Scientists are theorizing how to explain what happened during this time. In order to do so, they will have to find a law of physics to unify gravity, relativity and quantum mechanics (the behavior of matter on the scale of atoms or subatomic particles). This extremely brief period serves as an important milestone because it is only after this moment that we can explain the evolution of our universe.
10-43 to 10-35 second after the Big Bang: Even within this tiny span, known as the Grand Unified Theory (GUT) Era, major changes take place. The most important event: Gravity becomes its own distinct force, separate from everything else.
10-35 to 10-32 second after the Big Bang: During this short snippet of time, known as the Era of Inflation, the strong nuclear force separates from the remaining two unified forces: the electromagnetic and weak. Scientists are still not sure how and why this happened, but they believe it sparked an intense expansion — or “inflation” — of the universe. Measurements of the expansion during this time are extremely hard to comprehend. It seems that the universe grew by some 100 million billion billion times. (That’s a one followed by 26 zeros.)
Things at this point are really strange. Energy exists, but light as we know it does not. That’s because light is a wave that travels through space — and there is no open space yet! In fact, space is so crammed full of high-energy phenomena right now that matter itself cannot yet exist. Sometimes astronomers refer to the universe during this time as soup, because it’s just so hard to imagine how thick and energetic it would have been. But even soup is a poor descriptor. The cosmos at this time is thick with energy, not matter.
The most important thing to understand about the inflation era is that anything that was just a little bit different before inflation will become something that is a lot different later. (Hold on to that thought — it will be important shortly!)
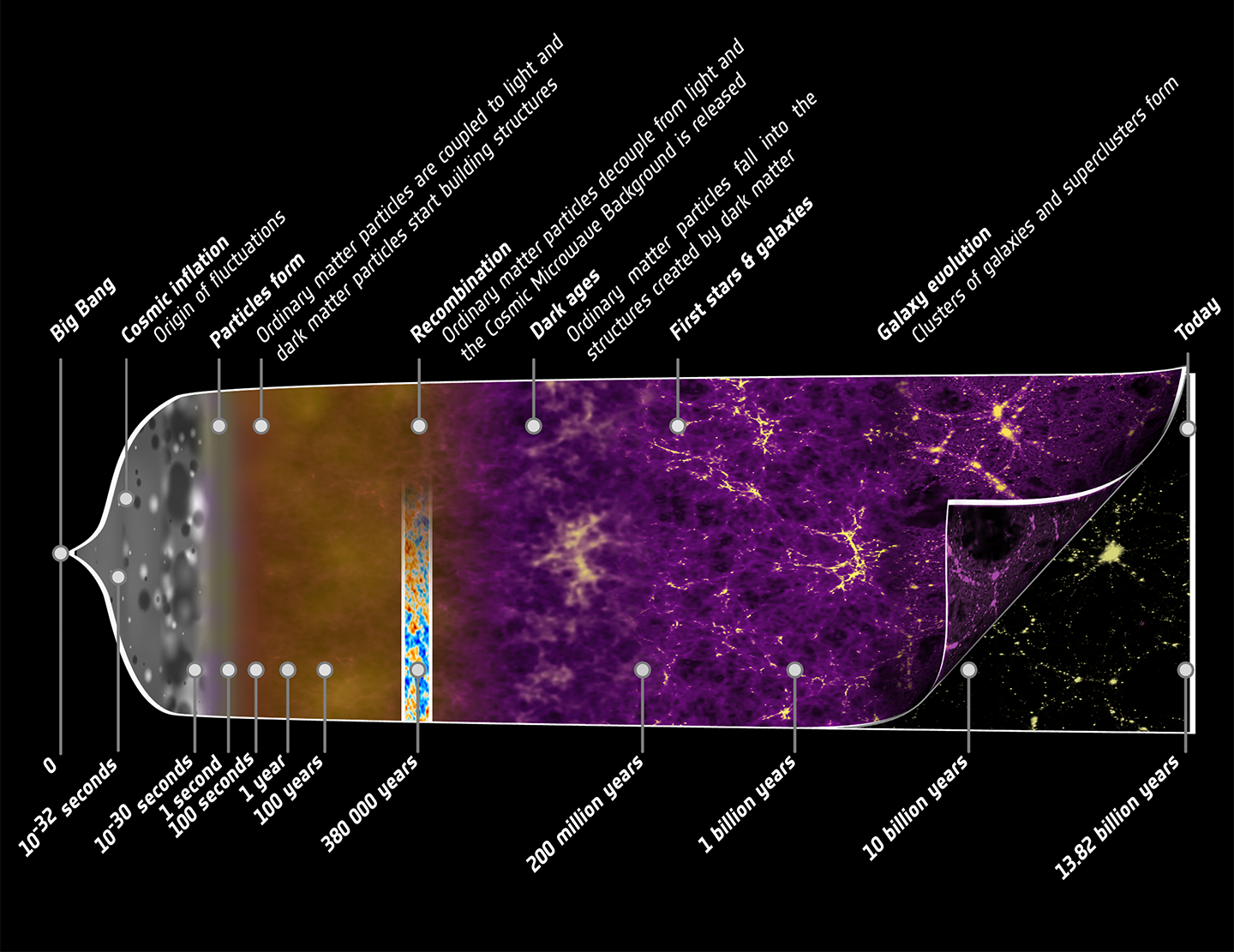
10-32 to 10-10 second after the Big Bang:
In this Electroweak Era, the weak force separates into its own unique interaction so that all four fundamental forces are now in place: gravity, the strong nuclear, weak nuclear and electromagnetic forces. The fact that these four forces are now independent lays the foundation for everything we now know about physics.
The universe is still too hot (too full of energy) for any physical matter to exist. But bosons — the subatomic W, Z and Higgs particles — have emerged as “carriers” for the fundamental forces.
10-10 to 10-3 (or 0.001) second after the Big Bang: This fraction of the first second is known as the Particle Era. And it’s full of exciting changes.
You probably have a photograph of yourself as a small child in which you start to see features that truly look like you. Maybe it’s a freckle that’s formed on your cheek or the shape of your face. For the cosmos, this transitional time — from the Electroweak Era to the Particle Era — is like that. When it’s over, some of the basic building blocks of atoms will finally have formed.
For instance, quarks will have become stable enough to combine to form elementary particles. However, matter and antimatter are equally abundant. This means that as soon as a particle forms, it almost immediately gets annihilated by its antimatter opposite. Nothing lasts for more than an instant. But by the end of this Particle Era, the universe had cooled enough to enable the next phase to start, one that moves us toward normal matter.
10-3 (0.001) second to 3 minutes after the Big Bang: At last we’ve reached a time — the Era of Nucleosynthesis — that we can really begin to wrap our heads around.
For reasons no one yet fully understands, antimatter has now become exceedingly rare. As a result, annihilations of matter and antimatter no longer happen as often. This allows our universe to grow almost entirely from that leftover matter. Space continues to stretch, too. The energy from the Big Bang keeps cooling off, and that lets heavier particles — like protons, neutrons, and electrons — begin to form. There’s still lots of energy all around, but the “stuff” of the cosmos has stabilized so that it is now almost entirely made of matter.
Protons, neutrons, electrons and neutrinos have become abundant and are beginning to interact. Some protons and neutrons fuse into the first atomic nuclei. Still, only the very simplest ones can form: hydrogen (1 proton + 1 neutron) and helium (2 protons + 2 neutrons).
By the end of the first three minutes, the universe has cooled so much that this primordial nuclear fusion comes to an end. It is still too hot to form balanced atoms (meaning, with positive nuclei and negative electrons). But these nuclei seal the makeup of our cosmos’ future matter: three parts hydrogen to one part helium. That ratio is still much the same today.
3 minutes to 380,000 years after the Big Bang: Notice that the timescales are now lengthening and becoming less specific. This so-called Era of Nuclei brings a return of the “soup” analogy. But now it’s a dense soup of matter: enormous numbers of subatomic particles including those primordial nuclei combining with electrons to become hydrogen and helium atoms.
The creation of atoms changes the organization of things considerably, because atoms hold together stably. Until now, “space” had hardly been empty! It had been packed full of subatomic particles and energy. Photons of light existed, but they would not have been able to travel far.
But atoms are mostly empty space. So at this incredibly important transition, the universe now becomes transparent to light. The formation of atoms literally opened up space.
Today, telescopes can look back in time and actually see energy from those first traveling photons. That light is known as the cosmic microwave background — or CMB — radiation. It’s been dated to roughly 400,000 years or so after the Big Bang. (For his study of how the CMB light serves as evidence for the cosmos’ current structure, James Peebles would share the 2019 Nobel Prize in physics.)
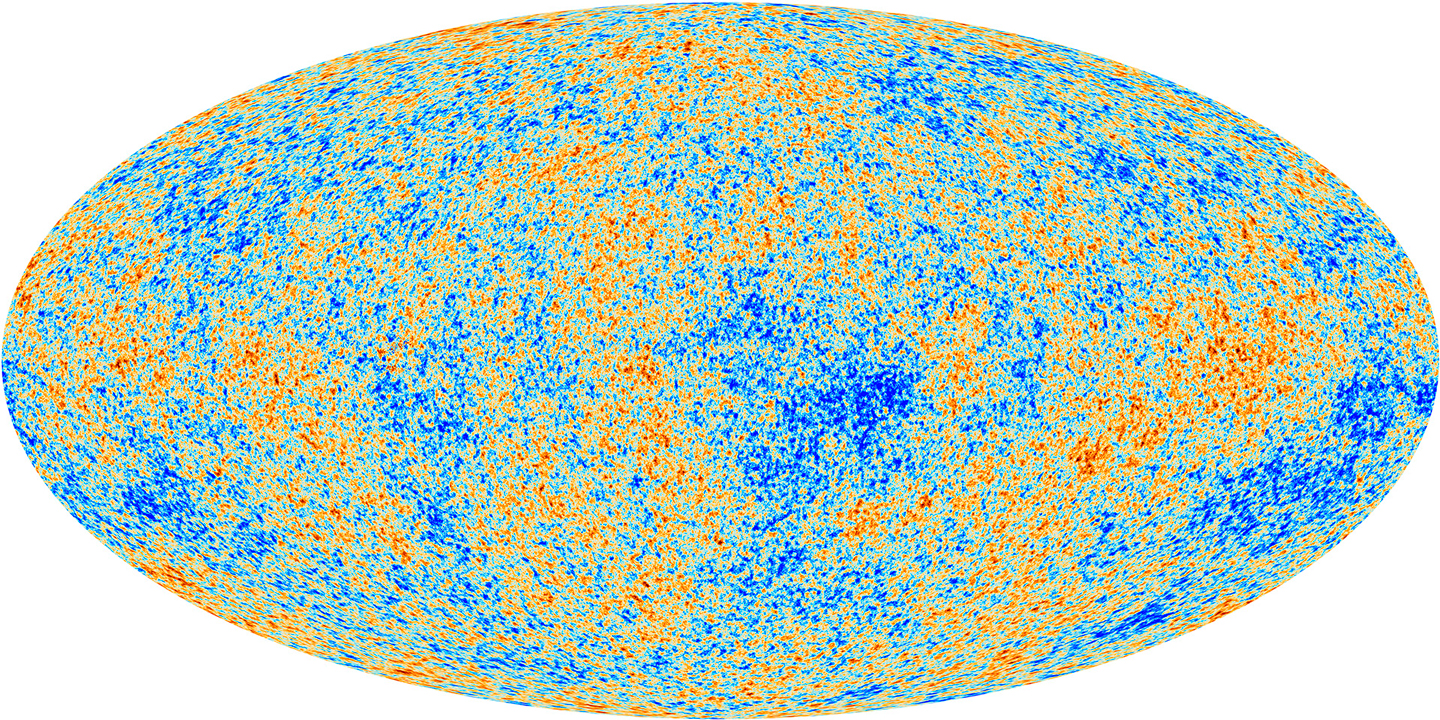
Space telescopes have measured this light. Among them are COBE (the Cosmic Background Explorer) and WMAP (the Wilkinson Microwave Anisotropy Probe). They measured the cosmic background temperature as 3 kelvins (-270º Celsius or -460º Fahrenheit). This background energy radiates from every point in the sky. You can imagine it as being like the warmth coming from a campfire even after it’s been extinguished.
The CMB wavelengths fall in the microwave portion of the electromagnetic spectrum. That means that it’s even “redder” than infrared light. As space itself has stretched during the expansion of the universe, the wavelengths of even the high-energy light from the Big Bang also have stretched. And it’s still there so that the right telescopes can see it.
COBE and WMAP discovered another amazing feature of the CMB. Remember that during the era of inflation, any tiny difference in the cosmic soup became magnified. The CMB radiation seen by COBE and WMAP indeed is almost exactly the same temperature everywhere across the sky. Yet these instruments did pick up tiny, tiny differences — variations of 0.00001 kelvin!
In fact, those temperature variations are believed to be the origin of galaxies. In other words, teeny tiny differences way back then became, over time — and as the universe cooled — the structures from which galaxies would begin to grow.
But that took time.
Redshift
As the universe has been expanding, the stretching of space has caused light to stretch as well, lengthening its wavelengths. This causes that light to redden. The James Webb Space Telescope is optimized to detect the faint, early — and now infrared — light from some of the oldest stars and galaxies.
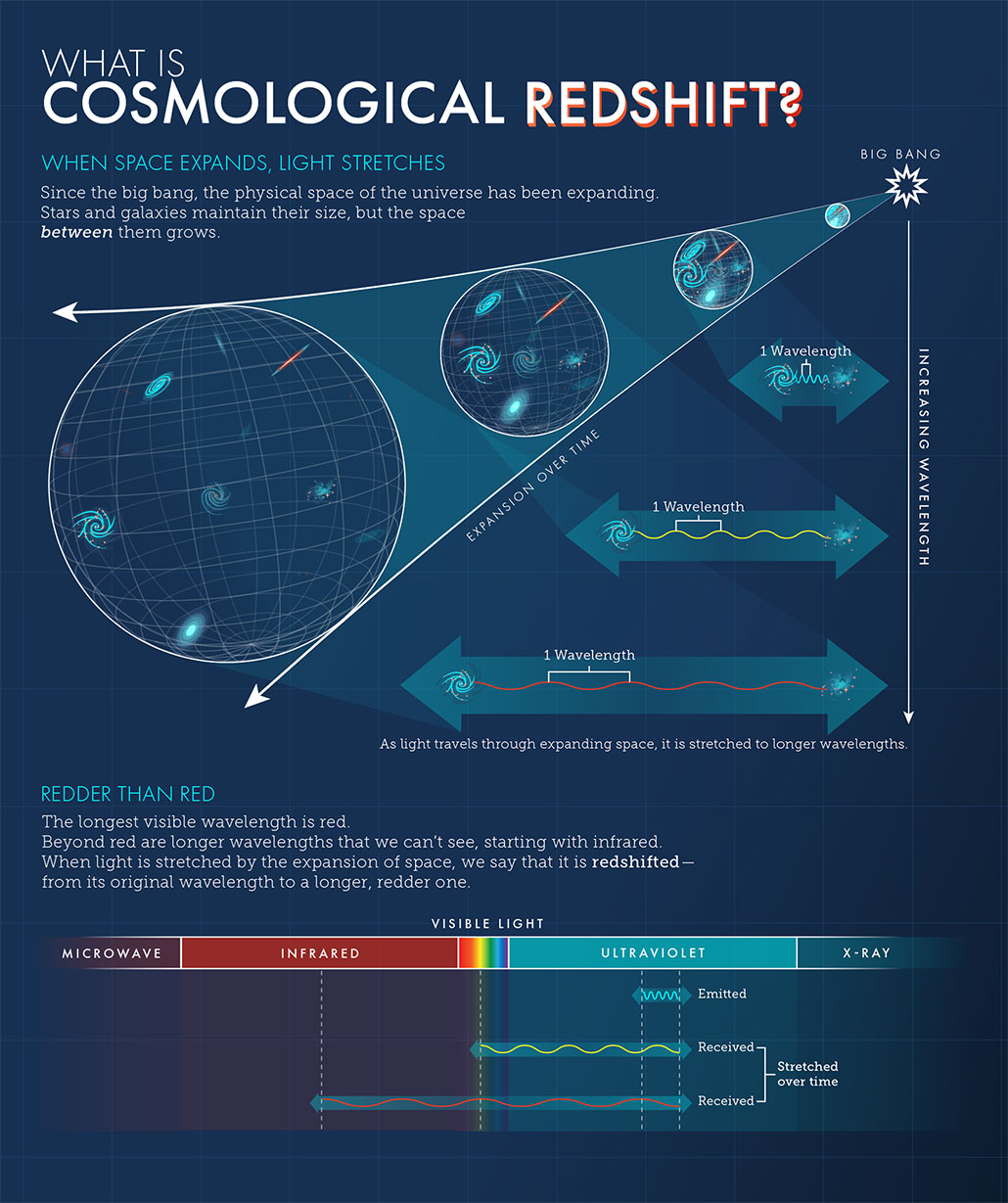
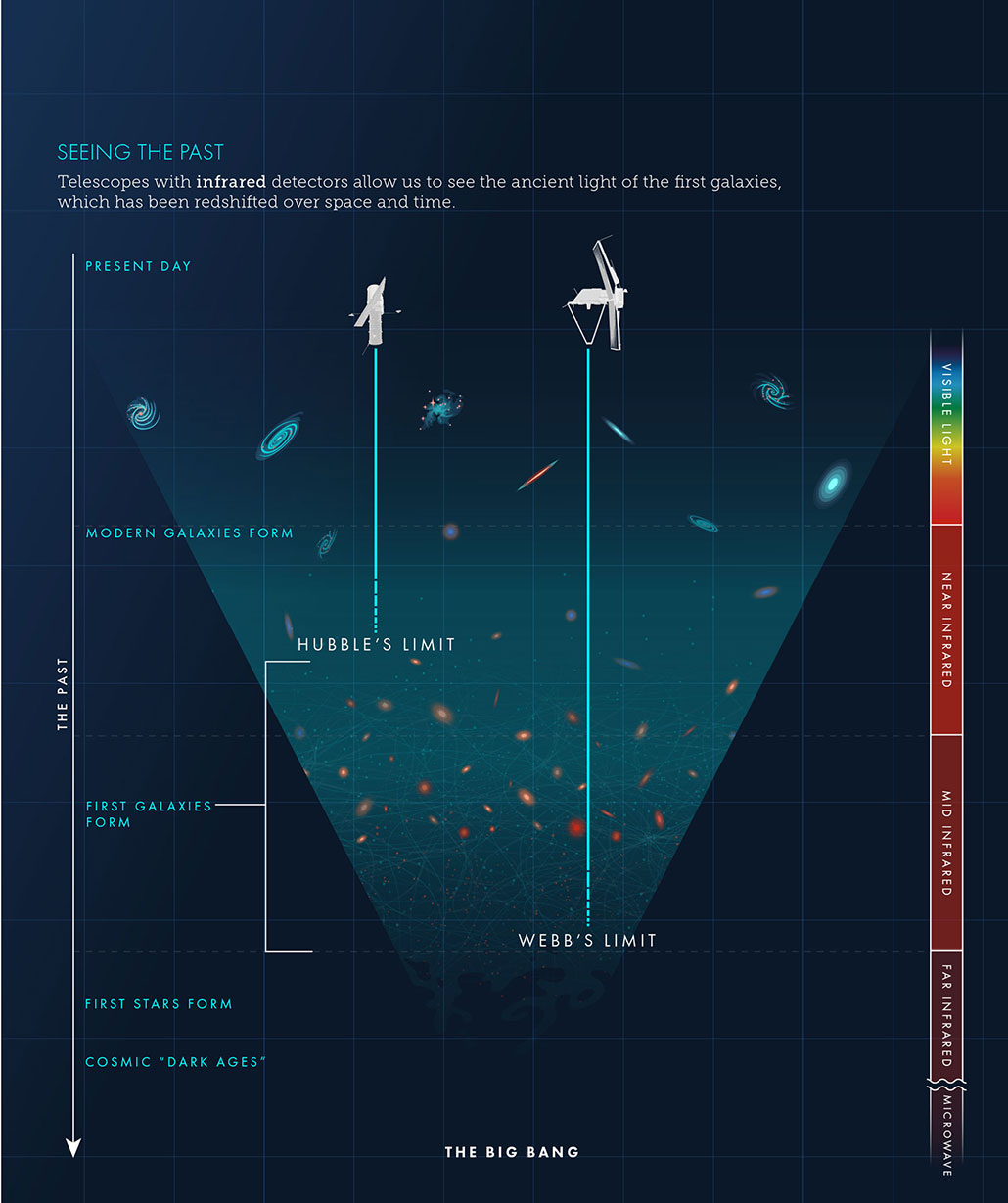
380,000 years to 1 billion years after the Big Bang: During this enormously long Era of Atoms, matter grew into the remarkable variety we now know. The stable atoms of hydrogen and helium slowly drifted together in patches, due to gravity. This further emptied space. And wherever the atoms clumped, they heated up.
This was a dark time for the universe. Matter and space had separated from each other. Light could travel freely — there just wasn’t much of it. As clumps of atoms grew both bigger and hotter, they eventually would start to spark fusion. It’s the same process that happened before (fusing hydrogen nuclei into helium). But now the fusion wasn’t happening everywhere, evenly. Instead, it became concentrated in the newly-forming centers of stars. Baby stars fused hydrogen into helium — then (over time) into lithium, and later still into the much heavier elements such as carbon.
Those stars would generate more light.
Throughout this Era of Atoms, stars began to fuse hydrogen and helium into carbon, nitrogen, oxygen and the other light elements. As stars grew older, they became able to exist with more mass. This, in turn, spawned heavier elements. Eventually, stars were able to burst beyond their previous bounds into supernovas.
Stars also began to attract one another into clusters. Planets and solar systems formed. This gave way to the evolution of galaxies.
1 billion years to the present time (13.82 billion years after the Big Bang): Today, we are in the Era of Galaxies. Only within the tiniest fraction of cosmic time have humans existed. Today, we see beautiful images of galaxies, stars, nebulae and other structures studded across the sky. We can see that there are patterns to where these structures end up; they are not evenly placed, but instead clump.
Every particle of matter continues to evolve, from the smallest scale of atoms to the largest scale of galaxies. The universe is dynamic. It changes, even now.
This cosmic scale of time remains hard to comprehend. But science is helping us understand it. And when we look deeper into space, as we are with the James Webb Space Telescope, we see farther back in time — closer to when it all started.
Notably missing from this timeline . . . is a lot of stuff we can’t see or even detect at this time. According to what physicists understand about the math of the universe, these other pieces are known as dark energy and dark matter. They could make up as much as a mind-boggling 95 percent of all the stuff in the universe. This timeline has only covered that roughly 5 percent of stuff that we know. How’s that for a Big Bang for your brain?